ENGN1215 Study Guide - Final Guide: Solid Solution Strengthening, Diamond Cubic, Crystalline Silicon
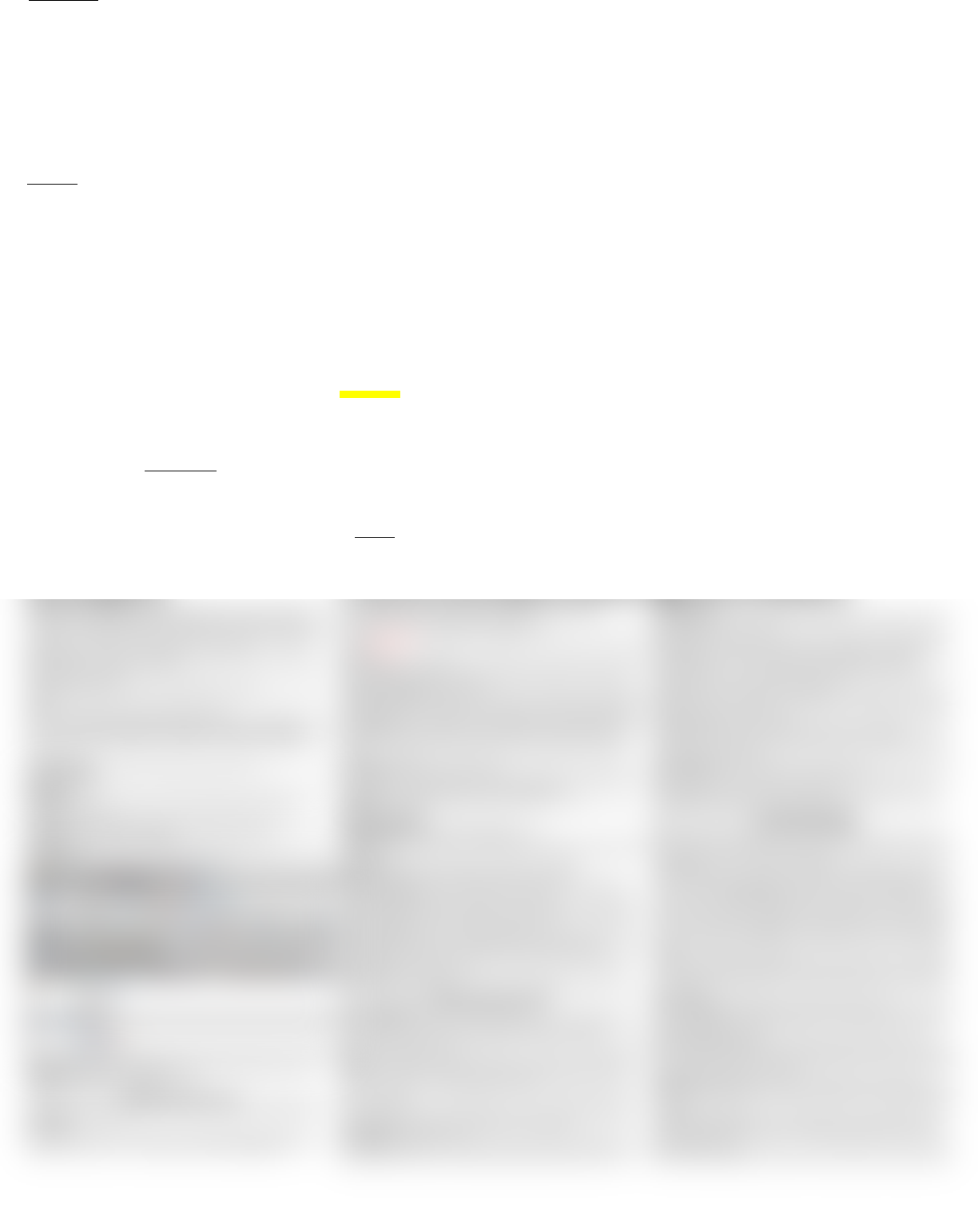
Mechanical properties:
RULE1: “The ability of a metal to plastically deform depends on the ability of dislocations to move”
RULE 2: “Restricting or hindering dislocation motion renders a material harder and stronger
M (schmid factor)= cosΦcosλ (Φ= angle between between slip plane and tensile axis, λ= angle between
slip direction and tensile axis) → most favoured has greatest schmid factor.
Τ (critical rescolved shear stress)= σ (applied stress) x m (scmid factor)
Slipping will not occur unless calculated applied stress is greater or equal to aplied stress given
High angle grain boundaries → harder for disloction to travel
Plastic deformation: Edge dislocation: dislocation moves in direction of applied stress. Screw dislocation:
Dislocation motion Is perpendicular to applied shear stress
Polycrystalline materials: Theoretically stronger than single crystal equivalents. Greater stress required to
initiate slip → (geometric constraint) grains cannot slip until other grains slip as well.Single crystals are better
in certain directions
Twinning: Can be fractional displacement. Occurs in BCC or HCP at low T/ high loading rate (slip is limited).
Good → introduces new slip system àmore slip possible
Strengthening mechanisms in metals:
Grain size reduction: This is based on the fact that it is difficult for a dislocation to pass into another grain,
especially if it is very misaligned. Atomic disorder at the boundary causes discontinuity in slip planes
Solid solution strengthening: Alloying with impurity atoms → substitutional or interstitial dislocations →
restrict dislocation movement. Can cancel dislocations out.
Strain hardening/ cold working: Hardening by plastic deformation. Increases dislocation density →
interacts with strain fields → dislocations hindered. Often reversible by heat treatment.
Recovery: Some internal stored energy relieved by dislocation movement.
Recrystallisation: Equiaxed, strain free, larger grains. Weaker, softer + more ductile . Heat treatment at
recrystallization T for 1 hour → completion.
Phase diagrams:
Free energy- function of internal energy of the system (enthalpy) + degree of randomness of atoms
(entropy).
Equilibrium → internal energy minimised.
Change in T→ changes energy state= no longer stable→ initiate change→ microstructure changes.
Phase compositon: single phase region the composition= same as the alloy
2 phae region= tie line to each boundary then drop down and composition L= percentage of stuff and comp
a= percentage of stuff.
Dotted lines=interdeterminate boundaries
Metastable system:having a state of apparent equilibrium although capable of
changing to a more stable state
Binary Isomorphous:two structures in solid phase.
If α, α+L, L → three phase.
When cooling occurs slowly atoms have time to diffuse and homogenize.
Non equilibrium cooling → cored grains (more of the high temperature element is retained at the centre
grains)
Solvus line: separates a homogeneous solid solution from several phases (limit of solubility)
Eutectic reaction: point at which material will change from one liquid to two solids and vice verca (usually
lowest melting T for system)
Eutectoid reaction: one solid into two different solids.
Peritectic reaction: two phase :liquid + solid transforms into a different solid
Terminal phase or terminal solution: is one that exists in the extremes of concentration (0 and 100%) of
the phase diagram
Intermediate phase: One that exists in the middle, separated from the extremes.
Intermetallic compound: precise chemical compositions. When using the lever rules, intermetallic
compounds are treated like any other phase, except they appear not as a wide region but as a vertical line.
Lamellar structure (alternating layers) forms at eutectic point → minimum diffusion distance i.e minimum
energy state.Gives alternating laminae layers of the two phases present.
In 2 phase materials to determine composition must draw a tie line(horizontal line extending to boundaries)
→ at boundaries construct vertical line to the compositional axis.
Lever rule: WL= (Cα–C0)/(Cα–CL, Wα= (C0–CL)/(Cα–CL).
Ferrite → BCC
Austenite → FCC, transforms into ferrite and cementite but volume change due to dif atomic packing
factors.
Cementite- (Fe3C) → very hard and brittle. Heated at 670ºC for several years → graphite.
Silicon accelerates this process .
Hypoeutectoid steel: carbon % below than eutectoid point. Hypereutectoid: carbon % greater than
eutectoid point.
Equilibium cooling= vertical line→ very slowly atoms have time to diffuse and homogenise
Phase transformations:
Types:
1. Simple diffusive (no. of phases remains constant) e.g. solidification of pure metals, allotropic
transformation, recrystallization/grain growth.
2. Complex diffuse (no. of phases changes) e.g. eutectoid reaction
3. Diffusionless metastable: e.g. martensitic transformation
Process:
1. Nucleation (submicron material of new phase material form in energetically favourable sites e.g. grain
boundaries)
2. Growth at expense of parent phase until energetic equilibrium reached
Kinetics of transformation: time dependence of transformation rate. In some cases cooling/ heating can
exceed transformation rate → supercooling/ superheating (nucleation does not have time to occur so
material remains liquid below freezing point until
homogeneous nucleation occurs → metastable)
Avrami eqtn: y=1-exp(-kt^n). k and n are time dep constants
Multiphase transformation: transformations caused by change in temp,pressure,composition etc
TTT diagram: time-temperature transformation diagram. At nose of this diagram transformation rate = max.
Proeutectic phase: Forms before reaching eutectic temperature. Specific to one composition. Requires
isothermal conditions (temperature stays constant)
Pearlite: ferrite + Fe3C (cementite): SLOW COOLING. Eutectoid reaction →.Carbon diffuses away from
the α regions into the cementite region. Forms when austentite is cooled below 723C. Bc transformation is
instantaneos normal difusive processes cannot be completed resulting in thin lamellae of the two
constituents. In theory structure= metastable in practice=permanent. Increased Fe3C → increased hardness
and brittleness. The lower the temperature the finer the pearlite structure. The faster the cooling the finer
the pearlite structure. Fine pearlite → more grain boundaries → harder and stronger. Coarse pearlite →
more ductile. At high T- diffusion rate = higher diffusion rate → coarser lamellae.
Bainite: MODERATE COOLING. Ferrite + cementite (very very fine, present in martensite matrix). Non
lamellar. Occurs at temp. Lower than pearlite formation.
Pearlite and bainite cannot exist together. Harder and strong ALSO ductile. Cooling rate: more rapid than
pearlite, less rapid than martensite
Spheroidite: heat pearlitic/bainitic steel at 700ºC or 24hrs. Lamella → spheres of
Fe3C in α matrix (Fe3C wants to minimise its surface area). Minimise grain boundaries. More ductile than
pearlite
Martensite: austenite rapidly cooled to ambient (RAPID QUENCH). Non
equilibrium single phase material. Diffusionless transformation of austenite. Hardest and strongest → most
brittle → due to it being in BCT phase(body centred tetragonal) lattice structure → lattice distortion and
hindrance . Forms almost instantaneously when the required low temperature is reached. Athermal
transformation (since no thermal activation is needed). REHEAT → Tempered martensite: Reduces internal
stress → increasing ductility and toughness without losing strength and stiffness. BCT, single phase →
ferrite + very
very small Fe3C particles.
Critical quenching rate: minimum quenching rate to give pure martensite structure
Athermal transformation: It forms nearly instantaneously when the required low temperature is reached;
since no thermal activation.
Metal alloys
Powder metallurgy: Compaction of metal + heat treatment àpowder granules fuse together. Used for
processing metals w. high melting temps bc process revolves around atomic diffusion between metal
particles rather than melting.
Forging:Make or shape a metal object by heating it in a fire or furnace and hammering it
Hot working:Processing metals above recrystallization temperature àmaterials can recrystallize during
deformation (no strain hardening àkeeps ductility high)
Cold working: shaping a metal below recrystallization temperature → strain hardening
Refractory metals:Metals that are extraordinarily resistant to heat and water e.g. alloys of molybdenum →
welding electrodes
Super alloys: superlative combinations of properties e.g. turbine blades
Refractory metals: e.g: tungsten, molybdenum, niobium, tantalum: addng these gives increase to all
properties. Used for llight filiments, welding elctrodes
Noble metals: gold, platinum, ruthenium, palladium- jewlellery
Superalloys: high performance alloy → aircraft turbine blades, nuclear reactor materials. Bc refractory
nature, chemical inertness, corrosion resistance
Structures and properties of ceramics
Structure: Made up of at least 2 elements. Crystal structures. Ionic or covalent. Crystal structure must be
electrically neutral. (+)Cations + anions(-) like to be in contact. Have well defined close packed planes
(usually large anions, small cations)
Structure types:
AX- no. cations = no. anions E.g. rock salt or Na+Cl-. Soluble in water but very high melt temp. due to
stability of interatomic bonds, (made up of two very reactive elements to form a stable one.) If ratio,rc/ra is
between .414 and .732 co-ord. no. is 6. This gives an FCC structure (2 interpenetrating FCC’s).
E.g. Cs+Cl- The CN number here is 8 and one to one atoms. Giving two interpenetrating simple cubic. Zinc
Sulphide, CN=4, diamond cubic tetrahedral.
AmXp- no. cations not equal to no. anions; Example is Calcium Fluoride of Ca2+F-. The CN=8.rc/ra=0.8 We
need twice as many fluoride then calcium. Still a simple cubic just less amount of calcium ½ to be exact.
Gaps in neighbouring cells. Ca will have a CN=4. Good for magnetism and electrical properties.
AmBnXp- several cations Here we have more than one type of cation present, so two metals and 1 non-metal.
E.g. Barium Titanate or Ba2+Ti4-2-, perovskite materials or energy producing materials. Usually in the form
AB 3 of the anion. All exist as FCC structures, but CN for Ba=12, Ti= 6, O=6.
Ceramic Density-
n= number of formula units in the unit cell, Aa = sum of atomic weights of all anions in formula unit, Ac= sum
of all atomic weights of all cations in formula unit, Vc= unit cell volume, Na= Avogadro’s number = 6.023e23
Silicate Ceramics- Silicate= silicon and Oxygen or Si4+ and O2-. It can mimic the organic nature of carbon
by bonding to itself. E.g. Salt, rock, clay, soil. The building block is 4 oxygen’s and 1 Silicon in a tetrahedron.
They are covalently bonded so it is a very negative building block (-4).
Silica Polymorphous- Material arranged in a 3-D network of tetrahedrons. Has a ratio of 1:2 (Si). So we
begin to get neutrality back globally. E.g. Quartz, brittle, low density very high melt temp. (1710°C).
Silica Glass Amorphous- Non-crystalline structure made up of very disordered units of (Si). Still tetrahedral
so consequently net negativity, Needs to be balanced by a positive element in the gaps, e.g.(Na+). The
functionality of glass is dependent on what cation you insert. Glasses are formed by adding elements in
oxide form, these are network modifiers. The oxides are absorbed but then oxygen separates. The additives
reduce viscosity. (High viscous mean thick).
Silicate Family- For an we can see that there is one oxygen in the middle bonded to 2 silicon’s so it is
stable however the three outer oxygen’s still have left over -1 charges so it has a net negativity of 6. Usual +
- layers.
Layers- The positive and negative layers form one on top of the other, being anion and cation layers. The
example of Kaolinite, positive and negatives are bonded in pairs, however the pairs of layers are weakly van
de wall bonded so interlayer cleavage is easy and hence slipping is easily achieved. That’s why we can
mould clay.
Firing Process- By heating it will bond the outer layers or outer layered pairs to form rigid structures. It will
drive off chemically bonded water, and give permanent shape. (Drying clay is not permanent.)
Polymorphism:ability to exist in more than on crystal structure e.g. crbo (diamond, graphite, fullerenes)
Diamond: metastable carbon polymorph. Formed underground under great stress. Hardest known element,
Low electrical conductivity, high thermal conductivity transparent, can use diamond coating to render
material hard.
Graphite: hexagonal carbon layers that are strongly bonded, Interlayering bonds (Van der Waals) weak,
Solid lubricant, Low thermal expansion → high thermal shock,High electrical conductivity parallel to sheets
Fullerenes (buckyballs): Distinct structure of discrete molecules of 60 atoms hexagonal and pentagonal
bonds in the shape of a sphere. FCC array. They are innately insulating but can be conductive if they are
alloyed. Due to the spare bonds In the structure they are quite reactive.
Types of defects: Defects in ceramics always occur in pairs to ensure electro neutrality is preserved.
Cation interstitial, Anion vacancy (too big for interstitial), Cation vacancy.
Must have +ve=-ve → electroneutrality → often pairs of defect (non-equilibrium situation are possible if
cation has more than one vacancy).
Frenkel defect- cation vacancy + cation interstitial-->- Cation moves causing an interstitial and leaving
behind a vacancy
Schottky defect- cation vacancy + anion interstitial Cation vacancy +Anion Vacancy, if you lose one the
other gets kicked out.
Properties
Fracture: Stress/strain behaviour detected with bending tests → generally zero plastic deformation (brittle).
Size dependant (bigger=weaker). Poor tensile strength, strong compressive strength → squishing of pores,
Porosity: Powder metallurgy is used to increase the density by eliminating pores. Porosity is far more
influential in brittle materials as opposed to ductile ones. Modulus of Elasticity decreases with the volume
fraction of porosity =modulus of elasticity of non-porous material. Flexural strength also decreases with
volume fraction of porosity. Porosity reduces cross sectional area where load is applied rendering it weaker.
E=E0(1-1.9P+0.9P^2) use this to work out stiffness. E= mod elasticity P= porosity
Glass:
Tg (transition temp.)- super cooled liquid → glass (from hard, glassy material to soft, rubbery material)
Strain point: fracture occurs before plastic deformation. Viscosity graph:
Annealing point: rapid atomic diffusion easy removal of residual stress.
Softening point: max. Temperature at which glass can be handled without significant shape change.
Melting point: fluid enough to mimic liquid.
Working point: point at which glass is easily deformed:
Glass forming: Must be pore free and homogenous → optical + strength
Gob and parison: A gob is a specific amount of molten glass, which is eventually formed. A parison is a
hollow and partially formed container that is formed in the blow mould. The paris on is then moved into a final
mould where bursts of high pressure gas mould to its final dimension.
Heat treating: Annealing it will heat it faster on the surface, when it is cooled thermal contraction occurs
which lead to residual stresses. These residual stresses need to be removed by heating beyond the anneal
point and slowly cooled.
Glass tempering: improves strength by introducing compressive residual surface stresses.Heat above
Tgbut below thermal softening point → cool to ambient with air/ oil jet.Causes hard surface but fluid centre →
contraction will cause inward stresses. To get it to a net position of zero stress is applied to overcome the
stress inside → very high strength in materials.
Composites- synergy of metals, ceramic and polymers so we can get properties from all of them.
Structural Basics- composed of a matrix phase and a reinforcement phase. One material inside the other.
Generally one will be stronger and harder and the other will be tough and ductile. e.g. wood
Composite Reinforcements- The fibres are the reinforcement phase and the properties they give are
influenced by, concentration, size of fibres, shape, distribution, orientation.
Particle Reinforced Composites- W e will have a matrix phase classified as a solvent and what is being
reinforced are the particles. Known as dispersion strengthening mechanisms. Most effective when particles
are uniformly distributed. Example, homogenising stones in cement mix.
Rule of Mixtures (STIFFNESS)- The stiffness of the material E is determined by the rule of mixtures,
E.g. For following constituents, calc the longitudinal and transverse stiffness of a continuous fibre composite
of fibre volume fraction 0.6: Carbon fibre - stiffness = 130GPa Epoxy resin - stiffness = 3GPa
Answer: Ef=130Gpa, Em=3GPa Vf=0.6 Gpa → Long= EmVm+EfVf =3(0.4)+130(0.6)=79.2 Gpa
Transverse=EmEf/VmEf+VfEm=3(130)/0.4(130)+0.6(3))= 14.61 Gpa.
(Vm =matrix volume fraction or 1-Vp, Em+Ep= matrix and particle stiffness)
Example, Tungsten particles in copper matrix this is a cutting tool and as more tungsten is applied the stiffer
the composite.
Example, Carbon Black in synthetic rubble which is essentially a car tyre. Carbon needs to be present so the
tyres don’t bounce.
Dispersion Strengthened Composites- particle reinforcement, much smaller scale so you can’t see them.
Similar to precipitation hardening where nano structures are included in the matrix. Very strong due to the
small precipitate. Each particle will be accompanied by lattice distortion making it hard. It is metastable
because as the precipitates grow it becomes useless.
Fibre Reinforced Composites- Strengthening or reinforcement of a relatively weak material by embedding
a stronger fibre phase within the weak matrix material. Most efficient technique due to the high surface area
to volume ratio (the success of a composite relies on its surface area). Usually a ceramic embedded into a
thermoset polymer. The most important part is the interface between the reinforcement and the matrix.
Fibre Length Effects- Fibrous composites work due to the ability to transfer load from the fibre to the matrix.
The fibre inputs high strength and stiffness → if a load is applied it will take the load and transfer it into the
matrix (transfer of mechanical energy) It will only transfer the load if it has a good interface and strong
chemical bond. The matrix will hold the fibre together until a load too big is applied and snaps along the
interface.
Lc or Efficient Fibre length-The fracture length is halfway and the length of the fibre is perfect so that
material isn’t wasted or snaps before max load is applied.
Fibre Orientation- Composites are highly anisotropic in the longitudinal direction vs the transverse direction.
If you load the fibre in the longitudinal direction it will take the stress and dissipate it through the matrix. To
fix this you layer it will something else in the other direction, hybrid composite.
Fibre Phase- Much stronger and more brittle then the matrix → take most of the load.
Matrix Phase- Used to dissipate the applied load evenly and protects the fibres, usually more ductile and
tougher than the fibre phase.
Types of Fibrous Composites
Polymer Matrix Composites- The matrix is ductile plastic matrix
Glass Fibre reinforced- Fibre Glass, used for car bodies and fishing rods.
Carbon Fibre- Black, very stiff and strong, the carbon fibre structure is complex and very strong it is used for
aerospace components, sports goods. Usually in an epoxy resin matrix. Quite brittle so aren’t load bearing.
E.g. sports goods
Aramid Fibre- KEVLAR it is tough strong and poor in compression. E.g. sports goods
Boron Fibre- Very stiff and niche in sporting goods, lighter and stiffer however toxic.
Metal Matrix Composites- Usually a ductile metal matrix (aluminium), they are often reinforced with
ceramic whiskers or refractory metals. Ceramics whiskers are very fine ceramic fibres, can be less than a
micron, although smaller the better, it is hard to align them e.g. aircraft alloys
Ceramic Matrix Composite- The matrix is a ceramic so it is brittle, Usually you have a ceramic ceramic
composition. By adding the reinforcement it improves fracture toughness. Example- PSZ in Al203 or Zr02.
Upon cracking and cooling it transforms from tetragonal to monoclinic, this will give a volume change that
supresses the crack growth, renders the ceramic tough and arrests the crack.
Structural Composites- These consist of sandwiches and laminates, Example is the aircraft panel floor.
Made up of honeycomb paper structure in the middle, then adhesive, then a composite panel or face sheet
that is very strong and light weight.e.g. Aircraft floor panels (sandwich, windshield (laminate)
Carbon-Carbon- A composite composed of overlapping continuous fibres of carbon imbedded in a carbon
matrix. It is extremely strong however very expensive. High tensile strength, high melt temp, works in a
heated environment, large fracture toughness.
Hybrid Composite- A composite that is fibre reinforces by two or more types of fibres, most common
example is glass fibres and carbon fibres layered on top of one another.) Higher impact resistance and not
anisotropic fractures. (Not catastrophic).
Corrosion and degradation of materials
Corrosion and Degradation- All materials degrade, it is very costly. Most pure metals want to corrode to
become thermodynamically stable, naturally only noble metals exist.
Corrosion in Metals- Corrosions is the destructive unintentional attack on metals by changing its very
being. Usually going from an atom to an ion. It involves the transfer of charges or a metal going into an ore.
Corrosion is a surface phenomenon and occurs at the interface of two entities. It is based on
electrochemistry and is the transfer of electrons between two chemical species. It can be either quick or slow
and occurs through oxidation and reduction.
Oxidation- This is classified as the loss of electrons M → Mn++ ne-. It gives up positively charged cations
and free electrons. Oxidation occurs at specifics at the surface, these sites are anodes or giving away sites.
Reduction-These free electron participate in a reduction reaction, whereby they will go to a cathode or
cathode site and the cathode site will take the electron or gain electrons.
Zn++ + 2e- → Zn. Or 2H++2e- → H2. This is hydrogen in solution or acid, where the hydrogens take the
electrons the metals give up. That’s why it fizzes when you put a metal in acid.Example. 02+4H++4e---> 2O
or O2+2O+4e---> 4(OH)-
Anodic and cathodic reactions occur simultaneously, and once it starts it will not stop. However because
they occur simultaneously you can pick part of the reaction to control, e.g. you can protect the anodic site
which will slow down the cathodic reaction, even when they are remotely separated.
Corroded metals: go into ionic solution or form insoluble salts. In electrochemical cell anode →corrodes,
cathode →grows (if in electrolyte same as metal)
Zinc Immersed in Acid- When you put the zinc in the acid it begins to bubble, this means corrosion is
occurring through ion movement. The zinc up the top is being eaten away by the acid reaction this is called
oxidation and termed an anodic site. It now has free elections and the zinc ions float off in solution. The
electrons no longer have a home anymore so go and form a cathodic site, the free electrons sit at the
surface and when the hydrogen ions float by they gain that electron to form a hydrogen atom. This is called
reductions and a cathodic reaction. The hydrogens then join to form molecules and bubbles which then float
off. (Process is called Effervescence.) Zn++ + 2e- → Zn. And 2H++2e-→ H2 Forms Zn+2H+→ Zn++ +. This
will continue until zinc or hydrogen run out.
Iron in Water- Corrosion of iron in water is a sow corrosion and causes rusting.
2Fe+02+2H2Oà2Fe+++4OH-à2Fe(OH)2 then 4Fe(OH)2+O2+2H2Oà4Fe(OH)3.
That final product is known as rusting and is the brown soluble material. Iron needs oxygen and water to
rust, if one is not present then it will not occur. Thus one of the best corrosion environments is oxygen is sea
water. However it sometimes doesn’t have oxygen present.
Electrode Potential- This is the corrosion susceptibility or reactivity of corrosion of material. Not all will
corrode at the same time due to difference electrode potentials. Whenever we have a multi metallic system
corroding there is one at a time. Closer to bottom of periodic table (lower V value) → more likely to under go
oxidation reaction → increasingly active. Overall cell potential: ∆V= V2-V1(V2is element undergoing reduction
reaction). Varies with T and solution concentration
Electrochemical Cell- Both materials sit in their ionic form solution, with a membrane separating them, the
membrane doesn’t let the solution pass only electrons. Thus causing conductivity. When they are connected
electrically it causes a corrosion system.
Example- The Fe electrode dissolves and oxidizes (anodic reaction) and the copper system grows (cathodic
reaction.)The electrons pass from the iron side to the copper side with a potential of 0.780. ie.
Cu+++FeàCu+Fe++. The more dissimilar the metals the larger the voltage. The materials are the electrodes
and the liquid is the electrolyte. It is called a Galvanic couple.
Example- Zinc is highly reactive so if we replace the copper with zinc the iron will now become the cathode
and the zinc is the anode. Consists of 2 standard half cells. Now electrons pass from zinc to iron with a
potential of 0.323V. Fe+++ZnàZn+++Fe.
Standard EMF Series- ranking of metallic elements according to their standard electrochemical cell
potentials. Reference standard half-cell → Hydrogen at 25ºC with inert Pt electrode → recorded voltage =
electrode potential → defines corrosionability (Hydrogen electrode has 0 voltage.)
The cell potential varies with T and the solution concentration.
To find the overall cell potential us the Nernst equation: F=96,500C/mol, R=8.314 gas constant, n=number of
electrons participating in the half cell electron or number of electrons the anodic material will lose.
T=Temperature. M1 and M2-Given concentrations, m1+m2n+ → m1n++m2
Finding cell potential: higher value-lower value on electrochemical series
Galvanic Series- This is a ranking system given for reactiveness of materials in seawater or marine
environments. Sea water is exceptionally corrosive compared to air, and is fatal for steel because of the
more amount of H+ ions and more chloride. The top most are unreactive and less corrosion susceptible and
the bottom are reactive. Some materials in the series are in brackets, to improve the materials corrosion
susceptibility you should alloy it with a material within that bracket. (Much more relevant scale.)
Corrosion Rates- The rate of removal=corrosion penetration rate (CPR), This measures how fast the
material will corrode and how far down. p=density, A=exposed area, higher area less corrosion, K-
87.6mm/year, W=weight loss after time t, T=time, N=number of electron, I=current, F=96500C/mol
If it has a big exposed area, the whole area will corrode but won’t go very deep. The depth is usually the
problem. This is why paint is bad because if it gets scratched there is a mall surface area and will corrode
deep. Therefore large anodes are very good.
Question: A nickel-based alloy has a 100nm thick oxide coating. Upon being placed in an oxidizing furnace
at 600/C for 1 hour, the coating has grown to 200nm in thickness. What will be the thickness after 1 day,
assuming a parabolic growth rate law? Answer: W^2=k1t+k2 → 3 values of t (0,1,24), 2 values of w (100 nm
and 200) use to find k1 and k2 then find new w.
Prediction or Overvoltage (n) - To predict the differences in performance over different conditions. It is the
degree of deviation from an equilibrium condition.
Example. Zn=-0.763V (ideal) in short circuited cell=-0.621V (not ideal condition).
Then n=-0.621—0.763=0.142V-this is the rate e would use in an open real world scenario.
Galvanic series gives reactivity’s in seawater → more relevant. Most pure metals want to corrode to
become thermodynamically more stable (not noble metals) : Rate of material removal = corrosion
penetration rate
Activation polarisation = when corrosion rate is controlled by slowest step. Example H2 on Zinc and
slowing one of these steps down
Concentration polarisation: diffusion of H+ to surface . The reaction rate of corrosion is limited by diffusion
in solution. E.g. if rate is low and/or concentration of hydrogen ions is high → adequate supply of ions at
surface. If not a depletion zone forms
Passivity- When a reactive metal becomes chemically inert. Aluminium is a good example because it
corrodes then stops. It gives a protective oxide layer on the surface which provides a protective barrier. As
soon as aluminium is exposed it will stop corroding. It will act very different in different environments, Steel
forms passive layer in acid, but corrodes in alkali. If passive layer is damaged it causes catastrophic
corrosion.
Switching Reactions- By applying an external voltage to the system we can make the anode act like the
cathode and vis versa. Where the solution will act like a cathode.
Corrosion Rates from Polarisation- When we plot an Electrochemical vs Current Density graph we will
get the gradients of anodic reactions and cathodic reactions, and the point at which it exists in equilibrium
equally cathodic and equally anodic. When we plot two Zinc and Hydrogen on one graph it will give an
intersection point where the two combine to give corrosion. The one downhill will be experiencing cathodic
reaction and the uphill will experience anodic reaction. Because zinc has a lower voltage it will corrode first
and faster because it has a larger current density, current density is a measure of how many electrons are
moving so the more that are moving the more corrosion will occur.
Concentration Polarization- If we have zinc (anode) in acid and we keep agitating the solution the
hydrogens will replenish and corrode the zinc completely, however if it is not agitate the cathode will incur
depletion whereby all hydrogens will be used up and are not replaced anymore forming a depletion zone.
Caused by stagnant electrolytes or mechanical barrier. They then go to plan B, if the anode or metal runs out
its fine but if the cathode runs out or hydrogens it will begin to use oxygen and chlorine to corrode which will
corrode from the inside out. The rate can be controlled by diffusion of H+ to the surface.
Note: If we had zinc on iron pillars in the ocean, we can plot zinc vs hydrogen and iron vs hydrogen. Iron will
be further up saying it needs more voltage so it will corrode last and the zinc will corrode before the pillar.
Tafel Plot- The straight line at the bottom means hydrogen has run out.
Forms of Corrosion:
Uniform Corrosion- Exposed to the environment and corroded uniformly. Example, copper roofs change
colour to green à leave behind a scale and deposit. Cathodic/anodic sites are completely random. Easy to
predict and design for. Prevention: coatings.
Galvanic Corrosion- Two metals/ alloys in a system they are electrically coupled to give a galvanic cell.
Most reactive will dissolve and the least reactive is immune. In the presence of an electrolyte in order for
conductivity to occur. Prevention: choose metals with similar electropotentials, choose favourable surface
area ratios (large anode surface area, small cathode surface area), insulate from one another.
Crevice Corrosion- Occurs in regions containing components of the same species. Caused by
concentration differences of ions/dissolved gases in electrolyte à depletion zone will occur and other ions will
be used to corrode (not uniform). H+ and Cl- dominate; oxygen is used up, inducing corrosion. Once a
depletion zone is formed it’s irreversible. Prevention: weld don't rivet
Pitting Corrosion- Pits of deep localised corrosion. Mechanism is the same as crevice corrosion, but will
occur when you have compositional differences in the material, i.e. alloys. (2 phase mixtures.) The electron
behaviours is very different, the difference in corrosion resistance in each element will cause one phase to
eat away the other. Prevention: add molybdenum.
Intergranular Corrosion- Occurs at the grain boundaries, which are high-energy states. Therefore when
corrosion has to be done it will start there. Especially if foreign substa nces are sitting at the
boundary.Example- Stainless steel that has been welded causes chromium and carbon to migrate to grain
boundary, these are hard precipitates, which weaken the boundary and cause depletion zones to occur.
Prevention: adding Ti/Nb and annealing.
Selective Leaching- Occurs in solid solution alloys. Corrosion where one element is preferentially dissolved
e.g. Zn from brass à weak/porous copper matrix. Prevention: protect surface
Erosion Corrosion- Very common in pipe work + turbine blades. Caused by fluid turbulence + hard
particles. The fluid gives a chemical attack and mechanical abrasion. Big problem in passivized material à it
removes protective layer. Prevention: design against fluid turbulence
Stress corrosion- combined action of corrosive environment and stress. Some materials inert until
stressed. Tends to lead to brittle fracture-even in ductile metals. E.g. stainless steal in a cl^- environment,
brass in a NH^3 environmentà cold work brassed pipes carrying ammonia.
Electrical properties of materials
Basics- The basics revolve around ohm’s law V=IR or resistivity=p=RA/l
Resistance is dependent on the physical size of a component, but Resistivity is a term you can look up in a
table. The reciprocal is conductivity, Conductivity=. Conductivity is the ability to pass an electric current.
Current density=J= =electric field intensity, essentially same as corrosion current density. There are 3 types
of materials, they are conductors, insulators and semiconductors.
Electric Current- results from the motion of electrically charged particles responding to forces applied from
external electric fields. Current can take the form of anything with a charge flowing. Most conduction is
caused by movement of electrons in an atom. Example sodium, has 1 electron in the valance band. It will go
and leave a hole behind as a charged entity, the amount of persuasion needed determines whether it is a
conductor or insulator. Conduction in Metals- The ability for a material to conduct is dependent on the
number of free electrons or how much energy we need to put into the system in order to excite it. The more
stable the system the more insulative. We need to input an energy to make it move and excite it to a higher
energy state. They leave a positive charge hole behind the same magnitude as the electron and the atom is
now an ion. It is easier when you have 1 valence electron and further out you are. The electro cloud also
needs exciting by external stimuli. Insulators- they are comprised of non-metals, It is a lot harder to excite
these electrons because the band gap is larger and more energy is required. You can make it conducting
however you will mechanically destroy it in the process. Band gap is large, narrow for semi, and negligible
for metals
Conduction in insulators and semi-conductors: Electrons are much harder to excite into an empty site.
Often need much more energy (usually thermal). Depends on energy band gap. Semi-conductors: band gap
= narrow. Insulators: band gap= high. Electrical resistivity: If temperature increases → thermal vibrations
increase → harder for electrons to get through → increased electrical resistivity. More impurity → higher
electrical resistivity
Electrical resistivity: If temperature increases → thermal vibrations increase → harder for electrons to get
through → increased electrical resistivity: More impurity → higher electrical resistivity
Intrinsic Semiconductors- Every excited electron will leave a hole, when the electron leaves they will both
travel in the opposite direction and in order for conduction to occur there must be no scattering centres, so
no impurities. Electrical conductivity depends on temperature and the band gap energy. Only silicon and
germanium will exhibit intrinsic behaviour.
Extrinsic Semiconductors- electrical conductivity is defined by the presence of impurities. Most
commercial semiconductor.Intrinsic- Characterised by a narrow band gap of less than 2eV. For each band
gap comes different properties. Two exist in pure form these are silicon and germanium. Taking an element
from group 3 and 5 or group 6 and 2 then you will get a good semiconductor. They are all characterised by a
different band gap conductivity and electron mobility.
Doping- adding small quantities of a foreign element to the system to give enhanced conduction.
n-type Extrinsic Semi conduction- Silicon has 4 electrons covalently bonded, if we replace one that has a
valance of 5 electrons one of them will move freely and is easily removed, It acts a substitutionally dopant.
E.g. Phosphorous. They won’t have a hole but just a cloud of electrons. This causes conductance along with
some holes.
p-type Extrinsic Semiconductors- This is using a valance of less than 4 (trivalent impurity) e.g. Boron. A
vacant site will form, which can be viewed as a positively charged hole. The hole then moves around
neighbouring silicon atoms taking their electrons causing conductivity. Example, crystalline silicon where
electrons are covalently bonded with each other. The split up and start roaming which cause conductivity.
(De coupling of atomic site.)
Effect of Temp. On Conductivity- For an extrinsic semiconductor doped with boron temperature does not
have much of an effect, but with an increase in boron is an increase in conduction. Intrinsic has a massive
find more resources at oneclass.com
find more resources at oneclass.com
Document Summary
Rule1: the ability of a metal to plastically deform depends on the ability of dislocations to move . Rule 2: restricting or hindering dislocation motion renders a material harder and stronger. M (schmid factor)= cos cos ( = angle between between slip plane and tensile axis, = angle between slip direction and tensile axis) most favoured has greatest schmid factor. (critical rescolved shear stress)= (applied stress) x m (scmid factor) Slipping will not occur unless calculated applied stress is greater or equal to aplied stress given. High angle grain boundaries harder for disloction to travel. Plastic deformation: edge dislocation: dislocation moves in direction of applied stress. Dislocation motion is perpendicular to applied shear stress. Polycrystalline materials: theoretically stronger than single crystal equivalents. Greater stress required to initiate slip (geometric constraint) grains cannot slip until other grains slip as well. single crystals are better in certain directions. Occurs in bcc or hcp at low t/ high loading rate (slip is limited).