Earth Sciences 2240F/G Lecture 8: Chapter 7
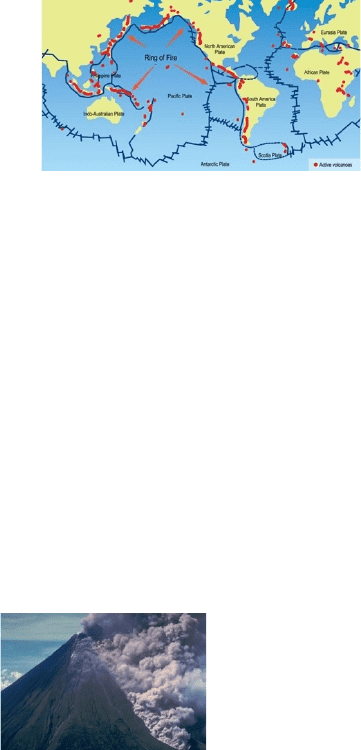
Chapter 7: Volcanoes- The Basics
1.0 Introduction
The second process we study that results from Earth’s internal energy is volcanic eruption. Dormant or extinct
volcanoes look great! There’s also a great deal of good that’s associated with volcanoes: many volcanic rocks
decompose very rapidly to form wonderfully rich agricultural soil; a large proportion of the world’s richest copper,
gold, and silver deposits are associated with volcanoes; and the heat associated with the deep magma and hot rocks
supplies a significant proportion of geothermal energy to consumers. Also, of course, the slopes of extinct volcanoes
are used in many parts of the world for recreational enjoyment such as down-hill skiing.
Volcanoes are testament to Earth’s huge internal energy, and the release of that energy can be catastrophic. Imagine a
volcanic eruption in which an area the size of Greater New York City collapses, a region the size of Nova Scotia is
suddenly buried under meters of hot ash that snuffs out all life, and farmer’s fields as far as 100 to 2000km away
(depending upon winds) are covered in so much ash that all crops are killed. Imagine that so much ash and gas is
blown into the atmosphere that the Sun’s light is dimmed to the point that there’s no summer for two years.
Unbelievable? It happened at least three times in the western USA alone when a huge volcano erupted at
Yellowstone National Park.
First we have to look at the source of energy and material, and consider a few ‘normal’ eruptions before we look at
the deadly ones.
Just as most (but not all) earthquakes are tied to current plate tectonics of Earth, most (but not all) volcanic eruptions
are also clearly tied to plate tectonics. In Figure 2 you see the strong correlation of plate boundaries and volcanoes.
Want to see an eruption? Well, while almost every day you can expect a new or continuing eruption around the rim
of the Pacific Ocean (due to the strong subduction of the Pacific Plate and the melting associated with it), for sure
there’s going to be eruptions at sea floor spreading centers (say, the Mid-Atlantic Ridge). Head for an island volcano
called Mt. Stromboli off the coast of Sicily; it erupts every 20 minutes to 1 hour every day!
2.0 Magma Characteristics
Magma is defined as molten rock, and may be a mix of hot liquid, gases, and scattered mineral crystals. When
magma spills out onto the Earth’s surface it produces hot lava (lava runs down the side of the volcano). When
magma blows out in a violent eruption, the stuff thrown into the air is usually called pyroclastic material (Fig. 4)
and may be in the form of blobs of hot magma (sometimes called ‘bombs’),very large solid blocks, or very fine
pulverized material commonly referred to (somewhat misleadingly) as ‘ash’. Initially, pyroclastic material may be
very, very hot because it is being blown out by very hot gases. All of the material erupted from a volcano forms
volcanic rock of various types when cooled and solidified. Magma can also solidify (cool and crystallize) beneath
surface (in which case it can’t be called ‘volcanic’), where it produces plutonic rocks. All types of rock produced
from magma– whether volcanic or plutonic- are classified as igneous rock.
Figure 4: Mount Mayon, Philippines
2.1 Viscosity and Volatiles
Viscosity is defined as that property of materials that provides resistance to flow; the opposite of viscous is fluid.
Whether or not a magma is viscous or fluid (or something in between) depends on the interaction of many
properties. But one factor over-rides all others: temperature– the higher the temperature of any material, the more
fluid (less viscous) it is. Reason: the higher the temperature, the greater the rate of atom vibration, thus bonds
between atoms (which would form structures in the liquid inhibiting fluidity) tend not to form very readily. In fact,
Figure 2: Active volcanoes and their locations in relation to
plate boundaries
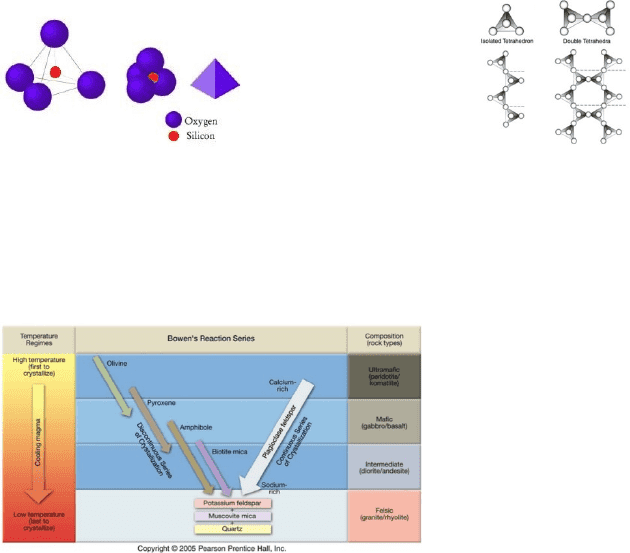
based on nothing but temperature, a liquid at 900°C would be about 100,000 times more fluid than the same liquid at
600°C.
Of course, there are other factors that influence viscosity; the next most important is chemical composition, in
particular the content of two elements, silicon (Si) and oxygen (O). Si and O atoms have a very strong affinity for
each other and tend to form small tetrahedral (pyramid) structures where each silicon atom is surrounded by 4
oxygen atoms (Fig. 5); the more of these little structures in a liquid, the more viscous the liquid. In addition, the
when tetrahedral are abundant they will tend to bond together to form long strings, sheets, and frameworks of Si and
O atoms by sharing corner atoms of those tetrahedral (Fig. 6). This can really quickly affect viscosity. Of course, the
tendency to form these silica tetrahedra is offset by temperature: few will form at high temperatures while a rapidly
increasing number will form as the magma cools.
Figure 5: Silica tetrahedral Figure 6: Chain silicates
If increasing the content of silica structures increases viscosity, magma with increasing content of crystals also
becomes increasingly viscous. As magma cools, more and more crystals form – eventually turning the material into
a solid rock. Take a look at Figure 7; start with a magma we’ll call basalt (defined later) that’s really hot and
contains no crystals, and now slowly cool it. The first crystals that form will be those that can form stable bonds
between atoms at high temperatures (olivine and pyroxene); at ever lower temperatures other minerals become
stable, until finally, at the lowest temperature of the magma (just before the whole thing becomes solid) quartz
(SiO2) forms.
There’s one final complication to the matter of viscosity, and that’s the volatile content of the magma– particularly
water since it’s by far the most common volatile compound. The water molecule is unique in that it is not
symmetrical; the two hydrogen atoms are bonded to the oxygen atom in such a way that one end of the molecule
(the hydrogen end) is relatively positively charged while the oxygen end is slightly negatively charged. We call this
a dipolar molecule.
Why is that significant? At moderate magma temperatures where silica tetrahedral would normally start forming and
making structures (where the oxygen of one tetrahedron shares as the oxygen of another tetrahedron and so on), the
slightly positive ends of water molecules attach lightly to the oxygen instead– thus breaking up the chain effect. The
result is to keep the magma more fluid than it would be if it contained no water. When water is attached to other
molecular structures in this way, it is called dissolved water. But there has to be a limit. You can readily understand
that if the magma happens to contain more water than there are opportunities for attachment to silica tetrahedral
structures that extra water will simply be left as bubbles of water– that is, exsolved water. It so happens that as the
temperature of the magma lowers, water is less able to attach to structures, and the amount of exsolved water
increases (we’ll see how that leads to explosions later).
2.2 Types of Magma
We can get a good idea of how the properties of magma control the type ofvolcanic activity we see by restrictingour
discussion to those magmas thatlead to three types of volcanic rock: basalt, andesite and rhyolite. Geologists go to
great lengths todetermine the source of a melt ormagma that produced particular sequences of rock. They have
determined that as far as volcanic rocksare concerned, nearly all magma hasbegun with a partial melt of Earth’s
asthenosphere; sometimes that partial melt is spewed out of a volcano with virtually no modifications to the initial
Figure 7: Bowen’s Reaction series showing the evolution of a
magma during cooling and the order in which minerals
crystallize.
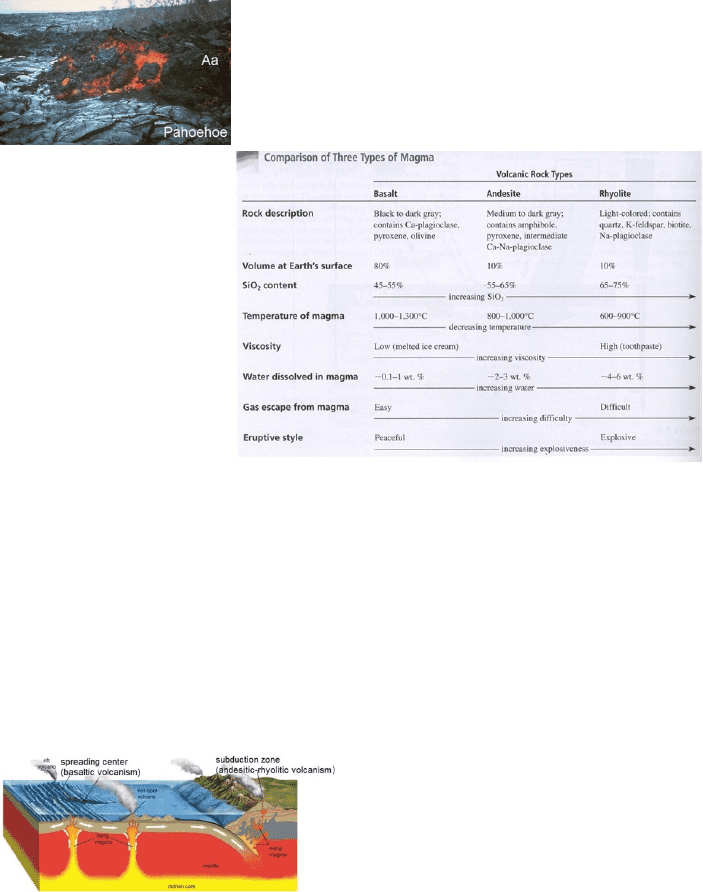
composition– that’s called basalt. Most basalt flows from a volcano as very hot and very fluid lava. There are two
basic textural types of basalt lava: aa (a rubbly-looking rock) and pahoehoe (a smooth-surfaced, ropey- looking
rock). Both are well-exposed in Hawaii, thus the Hawaiian names. The only significant difference in them is that aa
contains much less water than does pahoehoe.
Figure 9: Pahoehoe and aa lava
Andesite is volcanic rock produced from a modified partial melt. In other words, the primary melt that would
ordinarily yield basalt has been changed in composition somehow, the result being a magma that’s more silica-rich
and more volatile-rich than the original. Rhyolite is a volcanic rock even more modified (still higher silica and
volatile content) from the original partial melt that yields basalt. Figure 10 shows the essential differences in
resultant rock appearances and mineral content, in both chemical and physical properties of the magma, and finally
in the style of eruption expected from volcanoes fed by the different magmas.
Volcano Settings
Over 90% of volcanism is associated with edges of tectonic plates (look back at Fig. 2). Of the remaining 10%, most
is erupted from hot spots marking the tops of mantle plumes. Figure 10 is a hypothetical cross section through
Earth’s lithosphere and asthenosphere illustrating volcanic activity at a spreading center (left side) and at a
subduction zone (right side). We’ll look at those settings very briefly; we’re going to delay our discussion of
volcanic eruptions associated with mantle plumes until the next chapter.
Figure 11: Volcanism at spreading centers and subduction zones
3.1 Spreading Centers
Most active plate spreading takes place underwater. From the point of view of volcanic eruptions, mid-ocean ridges
constitute a comparatively simple environment (Fig. 11). As we learned in Chapter 3 (Plate Tectonics), at oceanic
spreading centers the asthenosphere– at temperatures usually between 1200°C and 1300°C– is as close as it ever
gets to the surface, and the cool lithosphere can be as thin as 3 km. As the plates diverge, the hot asthenosphere rises
toward the crest of the mid-ocean ridges. But at this point it is not melted. So how does a melt form from the hot
mantle/asthenosphere rock? The answer comes from Figure 12.