BIOL 4150 Lecture Notes - Lecture 8: Maximum Sustainable Yield, Mechanical Equilibrium, Ricker Model
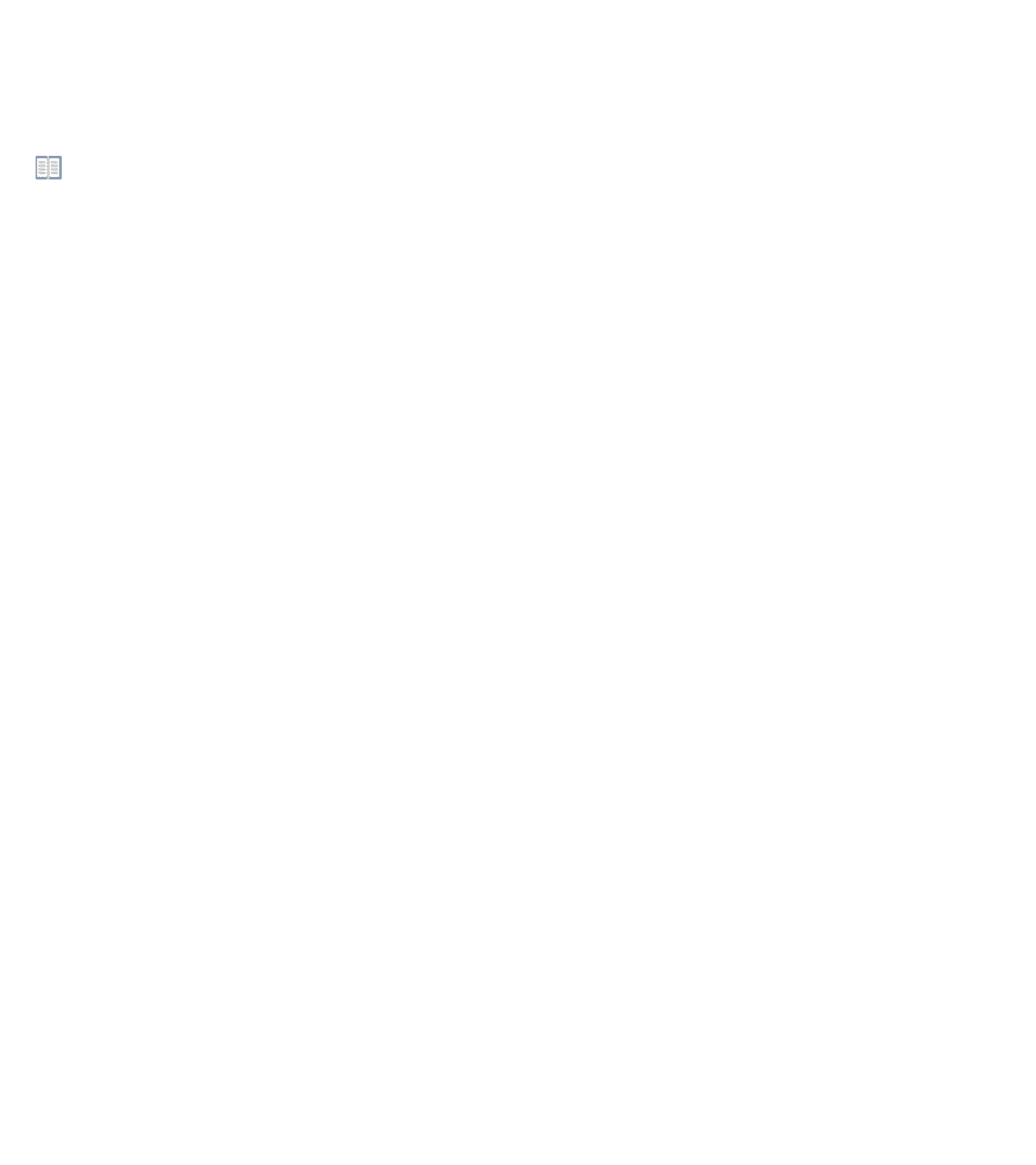
Chapter 19
11/02/17
Sustainable yield
•
Alternate harvesting strategies
•
Example: moose harvests in Ontario
•
Outline:
In some cases, man is the only significant predator
•
The long-term dynamics of such populations can be
modelled by assuming particular harvesting policies
•
Focus on 2 common policies: fixed quota vs. fixed
proportion harvesting
•
One of the major impacts on many populations is the
exploitation by man
f(N) = N* exp[rmax*(1-N/K)]
•
Creates hump shape function
○
Stable equilibrium is upper intercept of curve with
harvest number
○
At carry capacity, R(N) = 0
○
R(N) = f(N) -N --> net recruitment function
•
Ricker Logistic Model
Fixed quota harvest policies are predicated on sustained
use of the net recruitment, treating it as a surplus that can
be safely harvested without harming resource
sustainability in the long term
•
Points of equilibrium can be stable or unstable due
to differences in harvest and population size
○
Right: slight deviation --> followed by recovery
back to equilibrium (stable)
○
Population will either crash or grow
!
Left: slight deviation --> moves away from
equilibrium (unstable)
○
=maximum sustainable yield (risky!)
Apex of curve --> unstable equilibrium (harvest>
population)
○
Stable equilibrium vs. unstable equilibrium
•
Fixed Quota Harvest Strategy:
The total harvest is assumed to change with
population density, such that small populations will
yield lower harvests than large populations
○
The safest way to apply fixed mortality would be
to apply a constant harvest effort, but agencies
often have to use changing annual harvest quotas
that represent a target mortality level
○
General procedure is to control the level of mortality
exerted by harvesters, rather than having a fixed quota
removed from the resource population
•
q= proportion of the population harvested per unit
effort (catchability coefficient)
○
E(i) = effort level
○
Product = harvest mortality rate
○
H(N) = q*E*N
•
If population decreases, net recruitment is >
than harvest -->population will recover to
equilibrium
!
If population increases, net recruitment is
< than harvest -->population will decline to
equilibrium
!
Stable equilibrium at intercept of harvest mortality
rate and net recruitment function
○
Unstable equilibrium occurs at origin -do not need
to worry about it as much
○
Harvest mortality rate increases linearly with population
size
•
A conservation effort level might yield a similar
equilibrium harvest as more extreme effort, which
could encourage a more moderate policy
○
Both of these factors should tend to reduce the risk
of stochastic extinction events
○
Note: fixed proportion policies have a built-in mechanism
to reduce exploitation levels should resource density
decline to dangerously low levels
•
Fixed Mortality Harvest Strategy:
To manage moose population sustainably according to
cervid ecological framework
•
To provide an optimal mix of benefits from moose
through harvest allocation and related activities
•
rt= ln(Nt+T/Nt) /T
○
Carrying capacity: r=0
○
Growth rate decreases with increases population size
•
--> density dependent growth rates
•
Harvest proportion decreased with increasing
moose abundance
○
Have become more and more conservative
over time
!
Harvest effort decreased (smaller fraction)
○
Moose harvests fluctuate over time due to population size
•
*see time-dependent moose harvest mortality
•
Mean h = 11% of adult population
○
t=0 ---> t=20 has a decreased net recruitment rate
•
*see r-coding slide
○
If negative, Nt=0
!
N[t]<-max(result of model, 0)
○
Nt = Nt-1*exp(…)
!
H[t]<-min(h(Nt-1), Nt)
○
Constant mortality harvest model:
•
Stochastic due to environmental stochasticity
○
Harvest remains fairly constant
○
Rarely any excursions that result in population
threat
○
N vs. year
•
Intercepts recruitment curve at similar point of
fixed mortality
○
*see constant quota r-coding
○
More variable N but harvest is constant
○
Instead, use a quota (equivalent to proportion) = 0.02
•
Abundance is lower over time, more fluctuating
○
Harvest fluctuates a little
○
If h is raised to 0.3 (30%)
•
Population plummets to zero after year 50
○
Population is over-harvested so it collapses
○
Larger probability that stochastic factors will
cause population to decline
!
Q is too close to apex of net recruitment curve
○
Therefore, if constant quotas are used they must be
conservative (very small!)
○
If quota = 0.03
•
Example: Ontario Moose
In general, extinction risk is predicted to be higher in systems
with a fixed quota system than in those with a fixed mortality
system. The cost of this improved conservation, however, is
greater variability in harvests.
11/07/17
This can have interesting effects on the optimal
economic equilibrium and the risk of resource
exploitation
○
Market forces should influence the levels of harvest
effort exerted by commercial resource users
•
R(N) = f(N) - N
○
E = effort
!
q = catchability coefficient
!
Magnitude of slope = intensity that resource
is exploited
!
H(N) = q*E*N
○
--> fixed mortality
○
New equilibrium if effort or catchability is changed
○
When qE =rmax
!
Limit: tangent to recruitment curve
○
f(N) = N*exp[rmax(1-N/K)]
•
H = (H/qE)*exp[rmax(1-((H/qE)/K)] -(H/qE)
○
1 = (1/qE)*exp[rmax(1-((H/qE)/K)] -(1/qE)
○
qE = exp[rmax(1-((H/qE)/K)) - 1
○
--> Heq= q*E*K(1-(ln(1+qE))/rmax)
○
Substitute N=H/(qE) into H=N*exp[rmax(1-N/K)] - N
•
Equipment and gear
!
Time
!
People
!
Effort can include:
○
technology
!
Catchability can include:
○
Equilibrium harvests are maximized at intermediate
levels of effort
•
Bioeconomics:
As fishing intensity increases there is a peak in the catch
which then decreases
•
Costs(E)=cE
•
Revenue(E)=p*Heq(E) p=price
•
If effort is above this equilibrium, there is a deficit
costs>revenue
○
(Bio)economic equilibrium: when effort=cost
•
Ex. Tuna Fishing
When economics discuss cost, they usually mean opportunity
cost -the difference between the cost of one economic activity
vs. engaging in an alternative activity
Highest efficiency
•
Optimal effort is below maximum sustainable yield of
harvest
•
That is probably a good thing for conservation -it
implies that a monopolistic resource user ought to
choose relatively safe exploitation effort in order to
serve its own selfish needs
○
Note: that the level of effort that maximizes profits is
usually below the level of effort that maximizes
sustainable harvest
•
Incoming resource users won't necessarily care
about depressing the profit margin, so long as they
still find resource exploitation profitable
○
The problem arises when access to the resource industry
in unregulated
•
Presumably resource exploitation companies will want to
maximize profit
Sustainable but at risk --> sustainable and
abundant --> over-harvested and abundant -->
over-harvested and at risk
○
*see slide: utilization vs.biomass
•
As exploitation level increases, the biomass
(relative to MSY) decreases
○
As exploitation level decreases, the biomass
(relative to MSY) increases
○
Unsustainable by 1990
!
Over time, crosses MSY
○
Reduction in coastal fish catch rates over
time *Temperate Pacific (high probability of
collapse)
!
Expansion of coastal fish catch distribution over
time -spatial and temporal scales
○
Ex. Variation in fish biomass and exploitation rate over
time
•
Harvests often cycle over time to relative abundance
11/09/17
Harvest most intense on older, male animals
•
Sex-or age-selective harvesting can reduce the
demographic impact because reproductive capacity is less
affected
•
Social impacts of this can be counter-productive
•
Sex-or age-selective harvesting
3 different age classes for males and females
•
Number of deer grew exponentially over 6 years
○
Started with 4 female and 2 male
○
George Reserve deer herd
•
f0 = 1, f1 =1, f2=1 (fecundity)
○
s0 = 0.75, s1=0.75, s2=0.70 (survival)
○
Odd number rows = males
!
Even number rows = females
!
*create matrix
○
n = net vector
○
Geometric model:
•
*can have negative values, captures net
recruitment
!
Cannot use ricker model
!
A = M -I (transition matrix = raw matrix -initial
population)
○
n(t+1) = n(t) + [1 -(sum of n(t))/K]*A*n(t)
!
With density dependent effect:
○
Newborns > yearlings > adults
!
--> equal numbers of male and females (same vital
rates)
○
Logistic model:
•
Ex. Age-structured deer population
H -matrix
○
n(t+1) = n(t) + [1 -(sum of n(t))/K)*A*n(t) -
H*n(t)
○
Equivalent to net recruitment
!
--> harvest is scaled to population size
○
Logistic model with equal msy harvest:
•
Ex. Unselective deer harvest
Harvest is low compared to deer population
(harvest is lower)
○
Deer population: more reproducing individuals
(females)
○
Due to skewed ratio of male and females, a
population crash could be harder to recover from
○
Population almost reached carrying capacity
○
Logistic model with male adult-only harvest:
•
Ex. Sex and age-selective deer harvest
Hunt all males
•
*see slide
○
Logistic model:
•
Harvesting fewer females than males can lead to
increased yield and lower risk of collapse
•
--> don’t need as many males and females in the
population (not shown in model: no interaction
between sexes)
○
Males compete with females --> must max harvest
○
Generates the highest returns
•
Ex. Sex-selective deer harvest
Trade-off for hunters: limited number of adult males vs. less
meat on fawns
Proportion of females fecund dropped when
there was very few males to mate with
!
E.g. Russian saiga antelope
○
*to model, must include sex ratio
○
If male-biased harvest is extreme, it can lead to
reproductive collapse
•
Selective hunting can cause highly skewed sex ratio
Mean weight and horn length decreased with
increasing hunting effort
○
Therefore, rams that would ordinary be "the
most fittest" would not live very long and
therefore do not produce many lambs
!
Hunters selectively harvest rams of high potential
fitness
○
Ex. Harvesting effect on weight and horn length in
mountain sheep due to selection
•
*may be due to environmental changes rather than
human influences (e.g. vegetation, habitat,
competition)
○
There is declines over time in 11/17 antlered and 3/8
horned species
•
Human predation may be major agent of
selection
!
See rate of evolution due to harvesting vs. other
agents of natural selection
○
Rapid selection induced by harvesting could have
undesirable long term effects on sustainability (see slide)
•
Hunting effects on age of maturation and sexual ornamentation:
Harvesting and Population Dynamics
Thursday,+ November+ 2,+2017
11:32+AM
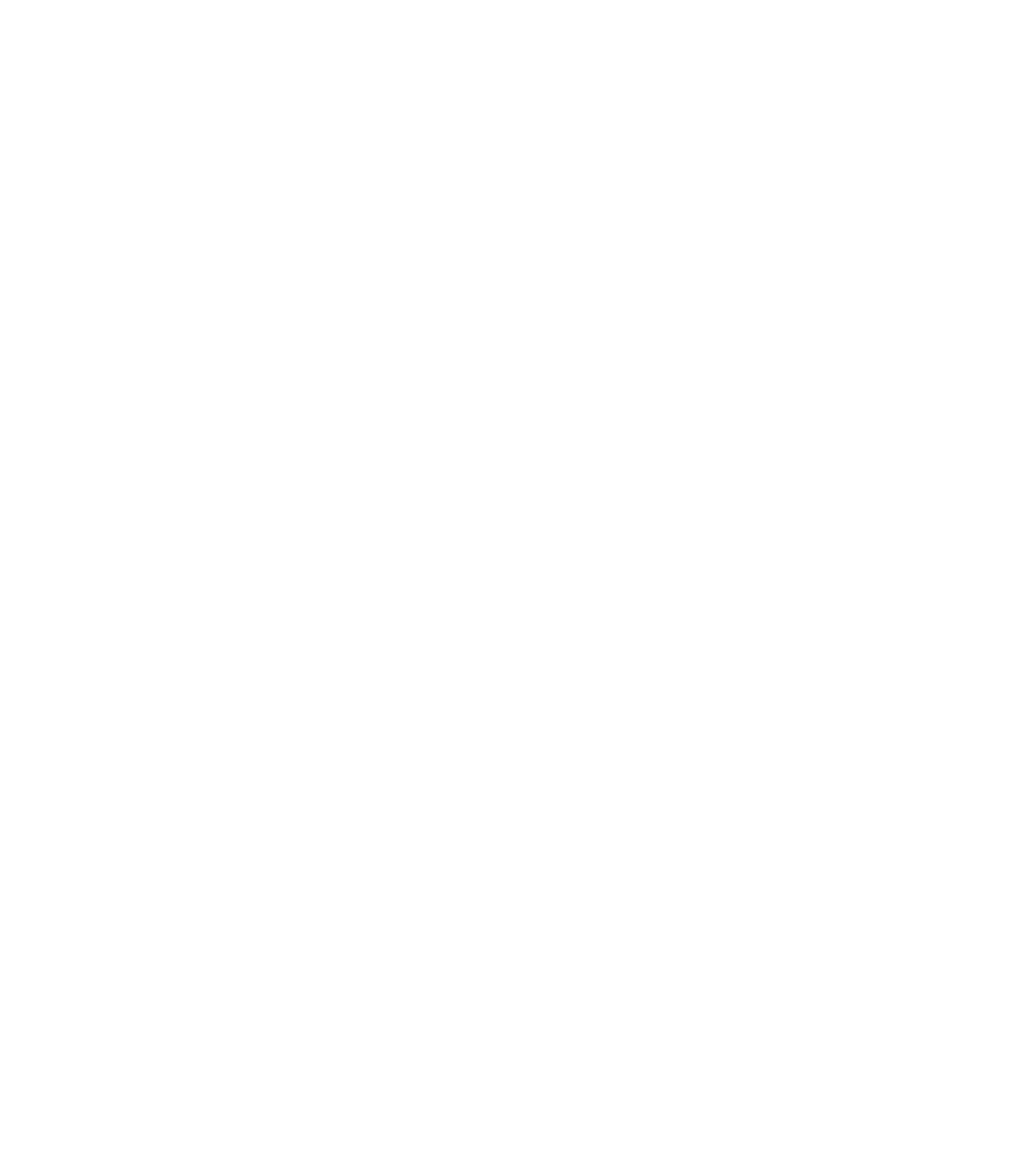
Chapter 19
11/02/17
Sustainable yield
•
Alternate harvesting strategies
•
Example: moose harvests in Ontario
•
Outline:
In some cases, man is the only significant predator
•
The long-term dynamics of such populations can be
modelled by assuming particular harvesting policies
•
Focus on 2 common policies: fixed quota vs. fixed
proportion harvesting
•
One of the major impacts on many populations is the
exploitation by man
f(N) = N* exp[rmax*(1-N/K)]
•
Creates hump shape function
○
Stable equilibrium is upper intercept of curve with
harvest number
○
At carry capacity, R(N) = 0
○
R(N) = f(N) -N --> net recruitment function
•
Ricker Logistic Model
Fixed quota harvest policies are predicated on sustained
use of the net recruitment, treating it as a surplus that can
be safely harvested without harming resource
sustainability in the long term
•
Points of equilibrium can be stable or unstable due
to differences in harvest and population size
○
Right: slight deviation --> followed by recovery
back to equilibrium (stable)
○
Population will either crash or grow
!
Left: slight deviation --> moves away from
equilibrium (unstable)
○
=maximum sustainable yield (risky!)
Apex of curve --> unstable equilibrium (harvest>
population)
○
Stable equilibrium vs. unstable equilibrium
•
Fixed Quota Harvest Strategy:
The total harvest is assumed to change with
population density, such that small populations will
yield lower harvests than large populations
○
The safest way to apply fixed mortality would be
to apply a constant harvest effort, but agencies
often have to use changing annual harvest quotas
that represent a target mortality level
○
General procedure is to control the level of mortality
exerted by harvesters, rather than having a fixed quota
removed from the resource population
•
q= proportion of the population harvested per unit
effort (catchability coefficient)
○
E(i) = effort level
○
Product = harvest mortality rate
○
H(N) = q*E*N
•
If population decreases, net recruitment is >
than harvest -->population will recover to
equilibrium
!
If population increases, net recruitment is
< than harvest -->population will decline to
equilibrium
!
Stable equilibrium at intercept of harvest mortality
rate and net recruitment function
○
Unstable equilibrium occurs at origin -do not need
to worry about it as much
○
Harvest mortality rate increases linearly with population
size
•
A conservation effort level might yield a similar
equilibrium harvest as more extreme effort, which
could encourage a more moderate policy
○
Both of these factors should tend to reduce the risk
of stochastic extinction events
○
Note: fixed proportion policies have a built-in mechanism
to reduce exploitation levels should resource density
decline to dangerously low levels
•
Fixed Mortality Harvest Strategy:
To manage moose population sustainably according to
cervid ecological framework
•
To provide an optimal mix of benefits from moose
through harvest allocation and related activities
•
rt= ln(Nt+T/Nt) /T
○
Carrying capacity: r=0
○
Growth rate decreases with increases population size
•
--> density dependent growth rates
•
Harvest proportion decreased with increasing
moose abundance
○
Have become more and more conservative
over time
!
Harvest effort decreased (smaller fraction)
○
Moose harvests fluctuate over time due to population size
•
*see time-dependent moose harvest mortality
•
Mean h = 11% of adult population
○
t=0 ---> t=20 has a decreased net recruitment rate
•
*see r-coding slide
○
If negative, Nt=0
!
N[t]<-max(result of model, 0)
○
Nt = Nt-1*exp(…)
!
H[t]<-min(h(Nt-1), Nt)
○
Constant mortality harvest model:
•
Stochastic due to environmental stochasticity
○
Harvest remains fairly constant
○
Rarely any excursions that result in population
threat
○
N vs. year
•
Intercepts recruitment curve at similar point of
fixed mortality
○
*see constant quota r-coding
○
More variable N but harvest is constant
○
Instead, use a quota (equivalent to proportion) = 0.02
•
Abundance is lower over time, more fluctuating
○
Harvest fluctuates a little
○
If h is raised to 0.3 (30%)
•
Population plummets to zero after year 50
○
Population is over-harvested so it collapses
○
Larger probability that stochastic factors will
cause population to decline
!
Q is too close to apex of net recruitment curve
○
Therefore, if constant quotas are used they must be
conservative (very small!)
○
If quota = 0.03
•
Example: Ontario Moose
In general, extinction risk is predicted to be higher in systems
with a fixed quota system than in those with a fixed mortality
system. The cost of this improved conservation, however, is
greater variability in harvests.
11/07/17
This can have interesting effects on the optimal
economic equilibrium and the risk of resource
exploitation
○
Market forces should influence the levels of harvest
effort exerted by commercial resource users
•
R(N) = f(N) - N
○
E = effort
!
q = catchability coefficient
!
Magnitude of slope = intensity that resource
is exploited
!
H(N) = q*E*N
○
--> fixed mortality
○
New equilibrium if effort or catchability is changed
○
When qE =rmax
!
Limit: tangent to recruitment curve
○
f(N) = N*exp[rmax(1-N/K)]
•
H = (H/qE)*exp[rmax(1-((H/qE)/K)] -(H/qE)
○
1 = (1/qE)*exp[rmax(1-((H/qE)/K)] -(1/qE)
○
qE = exp[rmax(1-((H/qE)/K)) - 1
○
--> Heq= q*E*K(1-(ln(1+qE))/rmax)
○
Substitute N=H/(qE) into H=N*exp[rmax(1-N/K)] - N
•
Equipment and gear
!
Time
!
People
!
Effort can include:
○
technology
!
Catchability can include:
○
Equilibrium harvests are maximized at intermediate
levels of effort
•
Bioeconomics:
As fishing intensity increases there is a peak in the catch
which then decreases
•
Costs(E)=cE
•
Revenue(E)=p*Heq(E) p=price
•
If effort is above this equilibrium, there is a deficit
costs>revenue
○
(Bio)economic equilibrium: when effort=cost
•
Ex. Tuna Fishing
When economics discuss cost, they usually mean opportunity
cost -the difference between the cost of one economic activity
vs. engaging in an alternative activity
Highest efficiency
•
Optimal effort is below maximum sustainable yield of
harvest
•
That is probably a good thing for conservation -it
implies that a monopolistic resource user ought to
choose relatively safe exploitation effort in order to
serve its own selfish needs
○
Note: that the level of effort that maximizes profits is
usually below the level of effort that maximizes
sustainable harvest
•
Incoming resource users won't necessarily care
about depressing the profit margin, so long as they
still find resource exploitation profitable
○
The problem arises when access to the resource industry
in unregulated
•
Presumably resource exploitation companies will want to
maximize profit
Sustainable but at risk --> sustainable and
abundant --> over-harvested and abundant -->
over-harvested and at risk
○
*see slide: utilization vs.biomass
•
As exploitation level increases, the biomass
(relative to MSY) decreases
○
As exploitation level decreases, the biomass
(relative to MSY) increases
○
Unsustainable by 1990
!
Over time, crosses MSY
○
Reduction in coastal fish catch rates over
time *Temperate Pacific (high probability of
collapse)
!
Expansion of coastal fish catch distribution over
time -spatial and temporal scales
○
Ex. Variation in fish biomass and exploitation rate over
time
•
Harvests often cycle over time to relative abundance
11/09/17
Harvest most intense on older, male animals
•
Sex-or age-selective harvesting can reduce the
demographic impact because reproductive capacity is less
affected
•
Social impacts of this can be counter-productive
•
Sex-or age-selective harvesting
3 different age classes for males and females
•
Number of deer grew exponentially over 6 years
○
Started with 4 female and 2 male
○
George Reserve deer herd
•
f0 = 1, f1 =1, f2=1 (fecundity)
○
s0 = 0.75, s1=0.75, s2=0.70 (survival)
○
Odd number rows = males
!
Even number rows = females
!
*create matrix
○
n = net vector
○
Geometric model:
•
*can have negative values, captures net
recruitment
!
Cannot use ricker model
!
A = M -I (transition matrix = raw matrix -initial
population)
○
n(t+1) = n(t) + [1 -(sum of n(t))/K]*A*n(t)
!
With density dependent effect:
○
Newborns > yearlings > adults
!
--> equal numbers of male and females (same vital
rates)
○
Logistic model:
•
Ex. Age-structured deer population
H -matrix
○
n(t+1) = n(t) + [1 -(sum of n(t))/K)*A*n(t) -
H*n(t)
○
Equivalent to net recruitment
!
--> harvest is scaled to population size
○
Logistic model with equal msy harvest:
•
Ex. Unselective deer harvest
Harvest is low compared to deer population
(harvest is lower)
○
Deer population: more reproducing individuals
(females)
○
Due to skewed ratio of male and females, a
population crash could be harder to recover from
○
Population almost reached carrying capacity
○
Logistic model with male adult-only harvest:
•
Ex. Sex and age-selective deer harvest
Hunt all males
•
*see slide
○
Logistic model:
•
Harvesting fewer females than males can lead to
increased yield and lower risk of collapse
•
--> don’t need as many males and females in the
population (not shown in model: no interaction
between sexes)
○
Males compete with females --> must max harvest
○
Generates the highest returns
•
Ex. Sex-selective deer harvest
Trade-off for hunters: limited number of adult males vs. less
meat on fawns
Proportion of females fecund dropped when
there was very few males to mate with
!
E.g. Russian saiga antelope
○
*to model, must include sex ratio
○
If male-biased harvest is extreme, it can lead to
reproductive collapse
•
Selective hunting can cause highly skewed sex ratio
Mean weight and horn length decreased with
increasing hunting effort
○
Therefore, rams that would ordinary be "the
most fittest" would not live very long and
therefore do not produce many lambs
!
Hunters selectively harvest rams of high potential
fitness
○
Ex. Harvesting effect on weight and horn length in
mountain sheep due to selection
•
*may be due to environmental changes rather than
human influences (e.g. vegetation, habitat,
competition)
○
There is declines over time in 11/17 antlered and 3/8
horned species
•
Human predation may be major agent of
selection
!
See rate of evolution due to harvesting vs. other
agents of natural selection
○
Rapid selection induced by harvesting could have
undesirable long term effects on sustainability (see slide)
•
Hunting effects on age of maturation and sexual ornamentation:
Harvesting and Population Dynamics
Thursday,+ November+ 2,+2017 11:32+AM
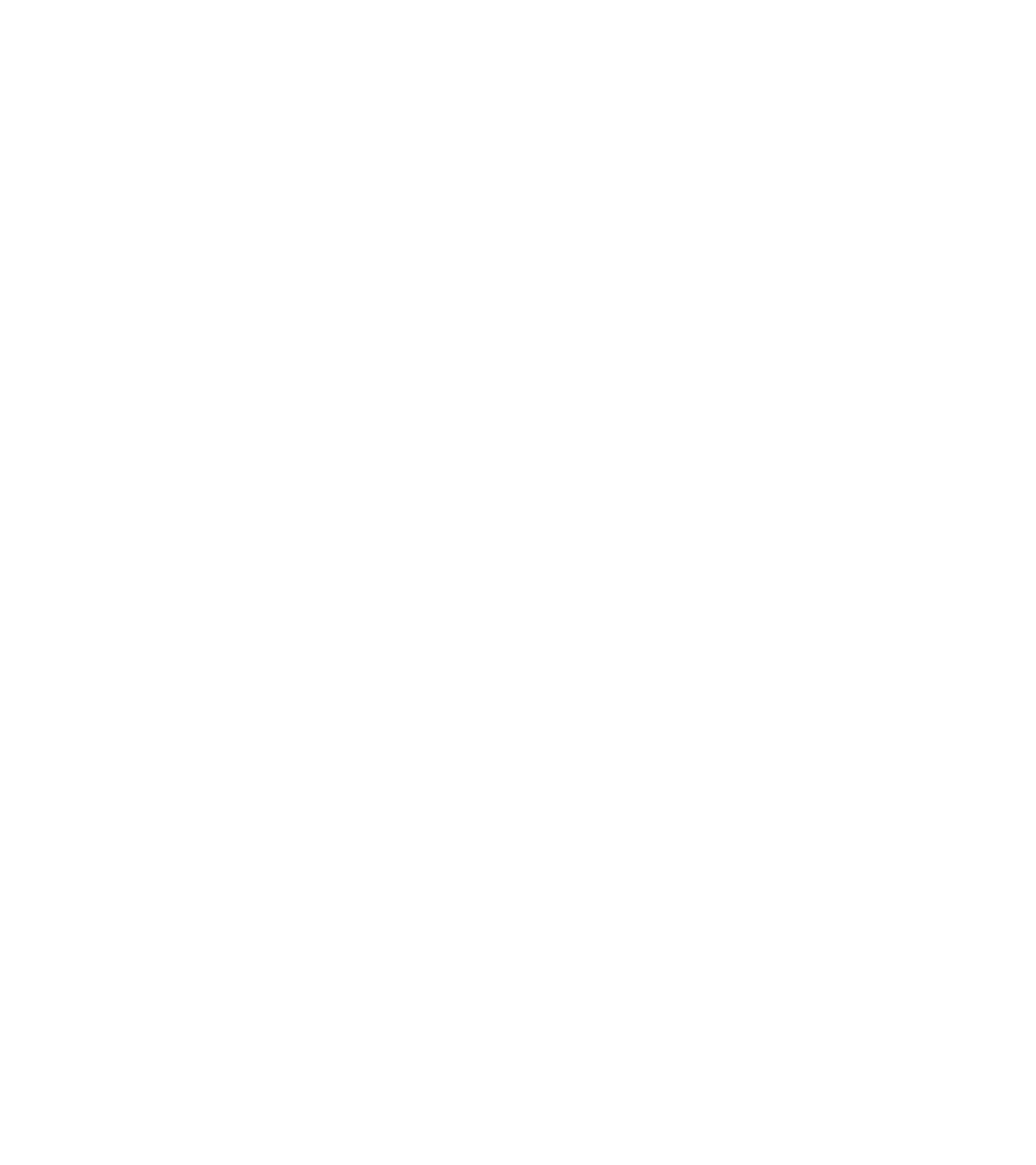
Chapter 19
11/02/17
Sustainable yield
•
Alternate harvesting strategies
•
Example: moose harvests in Ontario
•
Outline:
In some cases, man is the only significant predator
•
The long-term dynamics of such populations can be
modelled by assuming particular harvesting policies
•
Focus on 2 common policies: fixed quota vs. fixed
proportion harvesting
•
One of the major impacts on many populations is the
exploitation by man
f(N) = N* exp[rmax*(1-N/K)]
•
Creates hump shape function
○
Stable equilibrium is upper intercept of curve with
harvest number
○
At carry capacity, R(N) = 0
○
R(N) = f(N) -N --> net recruitment function
•
Ricker Logistic Model
Fixed quota harvest policies are predicated on sustained
use of the net recruitment, treating it as a surplus that can
be safely harvested without harming resource
sustainability in the long term
•
Points of equilibrium can be stable or unstable due
to differences in harvest and population size
○
Right: slight deviation --> followed by recovery
back to equilibrium (stable)
○
Population will either crash or grow
!
Left: slight deviation --> moves away from
equilibrium (unstable)
○
=maximum sustainable yield (risky!)
Apex of curve --> unstable equilibrium (harvest>
population)
○
Stable equilibrium vs. unstable equilibrium
•
Fixed Quota Harvest Strategy:
The total harvest is assumed to change with
population density, such that small populations will
yield lower harvests than large populations
○
The safest way to apply fixed mortality would be
to apply a constant harvest effort, but agencies
often have to use changing annual harvest quotas
that represent a target mortality level
○
General procedure is to control the level of mortality
exerted by harvesters, rather than having a fixed quota
removed from the resource population
•
q= proportion of the population harvested per unit
effort (catchability coefficient)
○
E(i) = effort level
○
Product = harvest mortality rate
○
H(N) = q*E*N
•
If population decreases, net recruitment is >
than harvest -->population will recover to
equilibrium
!
If population increases, net recruitment is
< than harvest -->population will decline to
equilibrium
!
Stable equilibrium at intercept of harvest mortality
rate and net recruitment function
○
Unstable equilibrium occurs at origin -do not need
to worry about it as much
○
Harvest mortality rate increases linearly with population
size
•
A conservation effort level might yield a similar
equilibrium harvest as more extreme effort, which
could encourage a more moderate policy
○
Both of these factors should tend to reduce the risk
of stochastic extinction events
○
Note: fixed proportion policies have a built-in mechanism
to reduce exploitation levels should resource density
decline to dangerously low levels
•
Fixed Mortality Harvest Strategy:
To manage moose population sustainably according to
cervid ecological framework
•
To provide an optimal mix of benefits from moose
through harvest allocation and related activities
•
rt= ln(Nt+T/Nt) /T
○
Carrying capacity: r=0
○
Growth rate decreases with increases population size
•
--> density dependent growth rates
•
Harvest proportion decreased with increasing
moose abundance
○
Have become more and more conservative
over time
!
Harvest effort decreased (smaller fraction)
○
Moose harvests fluctuate over time due to population size
•
*see time-dependent moose harvest mortality
•
Mean h = 11% of adult population
○
t=0 ---> t=20 has a decreased net recruitment rate
•
*see r-coding slide
○
If negative, Nt=0
!
N[t]<-max(result of model, 0)
○
Nt = Nt-1*exp(…)
!
H[t]<-min(h(Nt-1), Nt)
○
Constant mortality harvest model:
•
Stochastic due to environmental stochasticity
○
Harvest remains fairly constant
○
Rarely any excursions that result in population
threat
○
N vs. year
•
Intercepts recruitment curve at similar point of
fixed mortality
○
*see constant quota r-coding
○
More variable N but harvest is constant
○
Instead, use a quota (equivalent to proportion) = 0.02
•
Abundance is lower over time, more fluctuating
○
Harvest fluctuates a little
○
If h is raised to 0.3 (30%)
•
Population plummets to zero after year 50
○
Population is over-harvested so it collapses
○
Larger probability that stochastic factors will
cause population to decline
!
Q is too close to apex of net recruitment curve
○
Therefore, if constant quotas are used they must be
conservative (very small!)
○
If quota = 0.03
•
Example: Ontario Moose
In general, extinction risk is predicted to be higher in systems
with a fixed quota system than in those with a fixed mortality
system. The cost of this improved conservation, however, is
greater variability in harvests.
11/07/17
This can have interesting effects on the optimal
economic equilibrium and the risk of resource
exploitation
○
Market forces should influence the levels of harvest
effort exerted by commercial resource users
•
R(N) = f(N) - N
○
E = effort
!
q = catchability coefficient
!
Magnitude of slope = intensity that resource
is exploited
!
H(N) = q*E*N
○
--> fixed mortality
○
New equilibrium if effort or catchability is changed
○
When qE =rmax
!
Limit: tangent to recruitment curve
○
f(N) = N*exp[rmax(1-N/K)]
•
H = (H/qE)*exp[rmax(1-((H/qE)/K)] -(H/qE)
○
1 = (1/qE)*exp[rmax(1-((H/qE)/K)] -(1/qE)
○
qE = exp[rmax(1-((H/qE)/K)) - 1
○
--> Heq= q*E*K(1-(ln(1+qE))/rmax)
○
Substitute N=H/(qE) into H=N*exp[rmax(1-N/K)] - N
•
Equipment and gear
!
Time
!
People
!
Effort can include:
○
technology
!
Catchability can include:
○
Equilibrium harvests are maximized at intermediate
levels of effort
•
Bioeconomics:
As fishing intensity increases there is a peak in the catch
which then decreases
•
Costs(E)=cE
•
Revenue(E)=p*Heq(E) p=price
•
If effort is above this equilibrium, there is a deficit
costs>revenue
○
(Bio)economic equilibrium: when effort=cost
•
Ex. Tuna Fishing
When economics discuss cost, they usually mean opportunity
cost -the difference between the cost of one economic activity
vs. engaging in an alternative activity
Highest efficiency
•
Optimal effort is below maximum sustainable yield of
harvest
•
That is probably a good thing for conservation -it
implies that a monopolistic resource user ought to
choose relatively safe exploitation effort in order to
serve its own selfish needs
○
Note: that the level of effort that maximizes profits is
usually below the level of effort that maximizes
sustainable harvest
•
Incoming resource users won't necessarily care
about depressing the profit margin, so long as they
still find resource exploitation profitable
○
The problem arises when access to the resource industry
in unregulated
•
Presumably resource exploitation companies will want to
maximize profit
Sustainable but at risk --> sustainable and
abundant --> over-harvested and abundant -->
over-harvested and at risk
○
*see slide: utilization vs.biomass
•
As exploitation level increases, the biomass
(relative to MSY) decreases
○
As exploitation level decreases, the biomass
(relative to MSY) increases
○
Unsustainable by 1990
!
Over time, crosses MSY
○
Reduction in coastal fish catch rates over
time *Temperate Pacific (high probability of
collapse)
!
Expansion of coastal fish catch distribution over
time -spatial and temporal scales
○
Ex. Variation in fish biomass and exploitation rate over
time
•
Harvests often cycle over time to relative abundance
11/09/17
Harvest most intense on older, male animals
•
Sex-or age-selective harvesting can reduce the
demographic impact because reproductive capacity is less
affected
•
Social impacts of this can be counter-productive
•
Sex-or age-selective harvesting
3 different age classes for males and females
•
Number of deer grew exponentially over 6 years
○
Started with 4 female and 2 male
○
George Reserve deer herd
•
f0 = 1, f1 =1, f2=1 (fecundity)
○
s0 = 0.75, s1=0.75, s2=0.70 (survival)
○
Odd number rows = males
!
Even number rows = females
!
*create matrix
○
n = net vector
○
Geometric model:
•
*can have negative values, captures net
recruitment
!
Cannot use ricker model
!
A = M -I (transition matrix = raw matrix -initial
population)
○
n(t+1) = n(t) + [1 -(sum of n(t))/K]*A*n(t)
!
With density dependent effect:
○
Newborns > yearlings > adults
!
--> equal numbers of male and females (same vital
rates)
○
Logistic model:
•
Ex. Age-structured deer population
H -matrix
○
n(t+1) = n(t) + [1 -(sum of n(t))/K)*A*n(t) -
H*n(t)
○
Equivalent to net recruitment
!
--> harvest is scaled to population size
○
Logistic model with equal msy harvest:
•
Ex. Unselective deer harvest
Harvest is low compared to deer population
(harvest is lower)
○
Deer population: more reproducing individuals
(females)
○
Due to skewed ratio of male and females, a
population crash could be harder to recover from
○
Population almost reached carrying capacity
○
Logistic model with male adult-only harvest:
•
Ex. Sex and age-selective deer harvest
Hunt all males
•
*see slide
○
Logistic model:
•
Harvesting fewer females than males can lead to
increased yield and lower risk of collapse
•
--> don’t need as many males and females in the
population (not shown in model: no interaction
between sexes)
○
Males compete with females --> must max harvest
○
Generates the highest returns
•
Ex. Sex-selective deer harvest
Trade-off for hunters: limited number of adult males vs. less
meat on fawns
Proportion of females fecund dropped when
there was very few males to mate with
!
E.g. Russian saiga antelope
○
*to model, must include sex ratio
○
If male-biased harvest is extreme, it can lead to
reproductive collapse
•
Selective hunting can cause highly skewed sex ratio
Mean weight and horn length decreased with
increasing hunting effort
○
Therefore, rams that would ordinary be "the
most fittest" would not live very long and
therefore do not produce many lambs
!
Hunters selectively harvest rams of high potential
fitness
○
Ex. Harvesting effect on weight and horn length in
mountain sheep due to selection
•
*may be due to environmental changes rather than
human influences (e.g. vegetation, habitat,
competition)
○
There is declines over time in 11/17 antlered and 3/8
horned species
•
Human predation may be major agent of
selection
!
See rate of evolution due to harvesting vs. other
agents of natural selection
○
Rapid selection induced by harvesting could have
undesirable long term effects on sustainability (see slide)
•
Hunting effects on age of maturation and sexual ornamentation:
Harvesting and Population Dynamics
Thursday,+ November+ 2,+2017 11:32+AM
Document Summary
One of the major impacts on many populations is the exploitation by man. In some cases, man is the only significant predator. The long-term dynamics of such populations can be modelled by assuming particular harvesting policies. Focus on 2 common policies: fixed quota vs. fixed proportion harvesting. Ricker logistic model f(n) = n* exp[rmax*(1- n/k)] R(n) = f(n) - n --> net recruitment function. Stable equilibrium is upper intercept of curve with harvest number. Fixed quota harvest policies are predicated on sustained use of the net recruitment, treating it as a surplus that can be safely harvested without harming resource sustainability in the long term. Points of equilibrium can be stable or unstable due to differences in harvest and population size. Right: slight deviation --> followed by recovery back to equilibrium (stable) Left: slight deviation --> moves away from equilibrium (unstable) Apex of curve --> unstable equilibrium (harvest> population)