PHYS 182 Lecture Notes - Lecture 20: Redshift Survey, Dark Matter, Peculiar Velocity
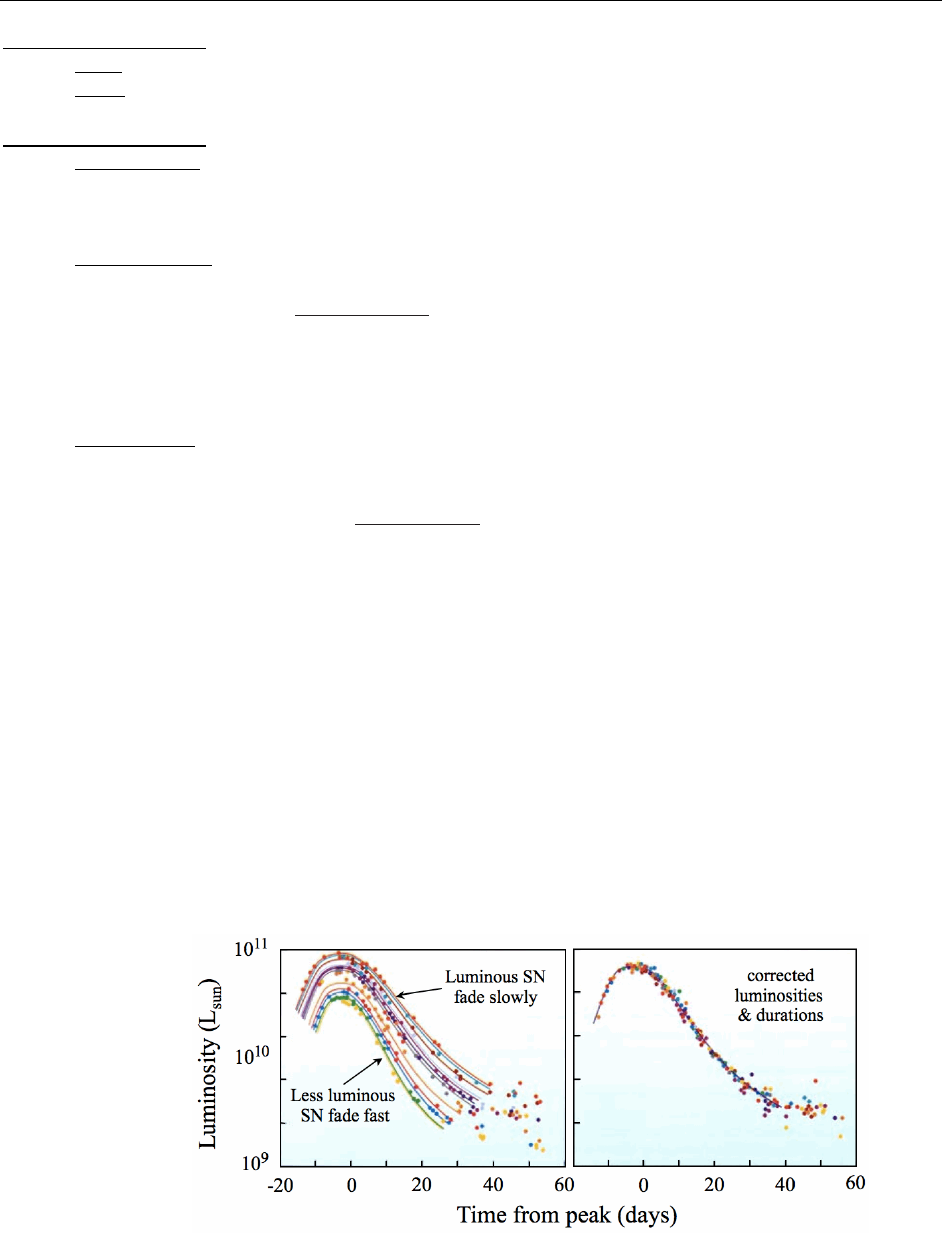
PHYS182: Our Evolving Universe
2017-11-21 LEC 20
10 - Galaxies & Beyond
- Goal: distribution of galaxies
- Need: Distance measurement
Distance measurement
1. Radar ranging: used to determine distances in the solar system with great accuracy
o Measure the time delay between the light pulses you emit (e.g. From Earth to Venus) and the
returning light pulse you receive
o Knowing the speed of light, from the time it takes you can get the distance
2. Parallax method: Used to determine distances near us within our own galaxy with great accuracy
o Using angles & geometry to accurately measure distance based on the parallax angle
o E.g. Sirius: p = 0.38”, d = 2.64pc
o This doesn’t work for objects that are so distant we can’t resolve the parallax angle
o Advantage: this method is independent of the properties of the star
3. Stellar candle: assume that objects we are looking at are all the same
o Masses & sizes vary and therefore are not stellar candles
o Use the relation between apparent & absolute brightness:
o Assume that luminosity is known (standard candle), you can measure apparent brightness
(B) and find the distance
i. B1/B2 = (d1/d2)2
o A) Cepheid stars: very luminous, pulsating
§ There is a relationship between period and luminosity
§ Fails at distances where you can’t make out individual stars
o B) Supernovae Type IA: Exploding white dwarves
§ You have mass falling into the white dwarf and if the mass of the white dwarf comes
to exceed the critical limit, they explode
§ There is a relation between decay time and absolute luminosity of the supernova
• Low luminosity supernova fade away faster than high luminosity supernova
§ So, from the time it takes to fade away, you can measure the absolute luminosity
• Determine the distance by measuring the apparent luminosity & using
formula above
§ Use this method to find distances of faraway galaxies
SURVEYING THE STARS
Measuring Apparent Brightness and Calculat-
ing Luminosity We can measure a star’s apparent bright-
ness by using a detector that records how much energy strikes its
light-sensitive surface each second. For example, such a detec-
tor would record an apparent brightness of 2.7 * 10-8 watt per
square meter from Alpha Centauri A (the brightest of the three
stars in the Alpha Centauri system). The only difficulties we face
in measuring apparent brightness are making sure the detector
is properly calibrated and, for ground-based telescopes, taking
into account the absorption of light by Earth’s atmosphere.
No detector can record light of all wavelengths, so any
measurement of apparent brightness applies only to some
small range of the complete spectrum. For example, if we use
a detector that is sensitive only to visible light, then our meas-
urement of a star’s apparent brightness allows us to calculate
only the star’s visible-light luminosity. Similarly, when we
observe a star with a spaceborne X-ray telescope, we measure
only the apparent brightness in X rays and can calculate only
the star’s X-ray luminosity. We will use the terms total lumi-
nosity (also called bolometric luminosity) and total apparent
brightness to describe the luminosity and apparent bright-
ness we would measure if we could detect photons across the
entire electromagnetic spectrum.
Note that the inverse square law for light works perfectly
only if the starlight follows an uninterrupted path to Earth.
In reality, the light of most stars passes through at least some
clouds containing interstellar dust on its way to Earth, and
this dust can absorb or scatter some of the star’s light. Today,
thanks largely to our modern scheme of stellar classification,
we can usually measure the effect of interstellar dust and
account for it when we apply the inverse square law for light.
A century ago, before astronomers knew of the existence of
interstellar dust, they often underestimated stellar distances
because they did not realize that the dust was making stars
appear less bright than they really are.
Measuring Cosmic Distances Tutorial, Lesson 2
Measuring Distance Through Stellar Parallax
The most direct way to measure a star’s distance is with stellar
parallax, the small annual shifts in a star’s apparent position
caused by Earth’s motion around the Sun. You can observe
parallax of your finger by holding it at arm’s length and look-
ing at it with first one eye closed and then the other. Astrono-
mers measure stellar parallax by comparing observations of
a nearby star made 6 months apart (FIGURE 3). The nearby
star appears to shift against the background of more distant
stars because we are observing it from two opposite points of
Earth’s orbit.
We can c al c ul ate a s tar ’s d is ta nc e if we kn ow t he p re ci se
amount of the star’s annual shift due to parallax. This means
measuring the angle p in Figure 3, which we call the star’s
parallax angle and is equal to half the star’s annual back-and-
forth shift. Notice that this angle would be smaller if the star
were farther away, so we conclude that more distant stars have
smaller parallax angles. Moreover, note that even the near-
est stars have parallax angles smaller than 1 arcsecond—well
below the approximately 1 arcminute angular resolution of
the naked eye—which explains why the ancient Greeks were
never able to measure parallax.
By definition, the distance to an object with a parallax
angle of 1 arcsecond is 1 parsec (pc). (The word parsec comes
from combining the words parallax and arcsecond.) Because
all stars have parallax angles smaller than one arcsecond, they
are all farther than 1 parsec away. If we use units of arcsec-
onds for the parallax angle, p, a simple formula allows us to
calculate distances in parsecs:
d
(in parsecs) =1
p
(in arcseconds)
For example, the distance to a star with a parallax angle of
1
2 arcsecond is 2 parsecs, the distance to a star with a parallax
angle of 1
10 arcsecond is 10 parsecs, and the distance to a star
with a parallax angle of 1
100 arcsecond is 100 parsecs.
Astronomers often state distances in parsecs, kiloparsecs
(1000 parsecs), or megaparsecs (1 million parsecs). However,
with a bit of geometry, it’s possible to show that 1 parsec is
equivalent to 3.26 light-years (see Mathematical Insight 2).
We can therefore modify the above formula slightly to give
distances in light-years:
d
(in light-years) =3.26 *1
p
(in arcseconds)
In this text, we’ll generally state distances in light-years rather
than parsecs.
Parallax measurement was the first reliable technique astro-
nomers developed for measuring distances to stars, and it
remains the only technique that tells us stellar distances without
any assumptions about the nature of stars. If we know a star’s
distance from parallax, we can calculate its luminosity with
the inverse square law for light. In fact, parallax measurements
FIGURE 3 Parallax makes the apparent position
of a nearby star shift back and forth with respect to distant stars
over the course of each year. The angle p, called the parallax angle,
represents half the total parallax shift each year. If we measure
p in arcseconds, the distance d to the star in parsecs is 1/p. The
angle in this figure is greatly exaggerated: All stars have parallax
angles of less than 1 arcsecond.
July
nearby star
distant stars
January
Every January,
we see this:
Every July,
we see this:
1 AU
p
d
Not to scale
As Earth
orbits the
Sun . . .
. . . the position of a
nearby star appears
to shift against the
background of
more distant
stars.
SURVEYING THE STARS
■ When we talk about how bright stars are in an absolute
sense, regardless of their distance, we are talking about
luminosity—the total amount of power that a star emits
into space.
You can understand the difference between apparent
brightness and luminosity by thinking about a 100-watt light
bulb. The bulb always puts out the same amount of light, so
its luminosity doesn’t vary. However, its apparent brightness
depends on your distance from the bulb: It will look quite
bright if you stand very close to it, but quite dim if you are
far away.
The Inverse Square Law for Light The apparent
brightness of a star or any other light source obeys an inverse
square law with distance, much like the inverse square law
that describes the force of gravity. For example, if we viewed
the Sun from twice Earth’s distance, it would appear dimmer
by a factor of 22 = 4. If we viewed it from 10 times Earth’s
distance, it would appear dimmer by a factor of 102 = 100.
FIGURE 2 shows why apparent brightness follows an
inverse square law. The same total amount of light must pass
through each imaginary sphere surrounding the star. If we
focus on the light passing through the small square on the
sphere located at 1 AU, we see that the same amount of light
must pass through four squares of the same size on the sphere
located at 2 AU. Each square on the sphere at 2 AU therefore
receives only 1
22=1
4 as much light as the square on the sphere
at 1 AU. Similarly, the same amount of light passes through
nine squares of the same size on the sphere located at 3 AU,
so each of these squares receives only 1
32=1
9 as much
light as the square on the sphere at 1 AU. Generalizing,
the amount of light received per unit area decreases with
increasing distance by the square of the distance—an inverse
square law.
This inverse square law leads to a very simple and impor-
tant formula relating the apparent brightness, luminosity, and
distance of any light source. We will call it the inverse square
law for light:
apparent brightness =luminosity
4p*distance2
Because the standard units of luminosity are watts, the units of
apparent brightness are watts per square meter. (The 4p in
the formula above comes from the fact that the surface area
of a sphere is given by 4p * radius2.)
In principle, we can always determine a star’s apparent
brightness by carefully measuring the amount of light per
square meter we receive from the star. We can then use the
inverse square law to calculate a star’s luminosity if we can
first measure its distance, or to calculate a star’s distance if we
somehow know its luminosity.
FIGURE 2 The inverse square law for light: The apparent bright-
ness of a star declines with the square of its distance.
The same amount
of starlight passes
through each
sphere.
The surface area
of a sphere depends
on the square of its
radius (distance
from the star) . . .
. . . so the amount
of light passing
through each unit
of area depends on
the inverse square
of distance from the
star.
1 AU 2 AU 3 AU
MATHEMATICAL INSIGHT 1
The Inverse Square Law for Light
Suppose we are located a distance d from a star with luminosity
L. The star’s apparent brightness is the power per unit area that
we receive at our distance, d, which we find by imagining a giant
sphere with radius d (similar to any of the three spheres in Figure 2)
and surface area 4π * d2. (The surface area of any sphere is 4π *
radius2.) All the star’s light passes through the imaginary sphere, so
the apparent brightness at any point on this sphere is the star’s lumi-
nosity, L, divided by the sphere’s surface area, which is the formula
we call the inverse square law for light:
apparent brightness =star>s luminosity
surface area of imaginary sphere
=L
4p*d2
EXAMPLE: What is the Sun’s apparent brightness in our sky?
SOLUTION:
Step 1 Understand: The Sun’s apparent brightness is the power per
unit area that we receive in the form of sunlight. We find this power
with the inverse square law for light, using the Sun’s luminosity
and Earth’s distance from the Sun; for unit consistency, we put the
Earth-Sun distance in meters.
Step 2 Solve: The Sun’s luminosity is LSun = 3.8 * 1026 watts, and
Earth’s distance from the Sun is d = 1.5 * 1011 meters. The Sun’s
apparent brightness is therefore
L
4p*d2=3.8 *1026 watts
4p*(1.5 *1011 m)2
=1.3 *103 watts/m2
Step 3 Explain: The Sun’s apparent brightness is about 1300 watts
per square meter at Earth’s distance.
THINK ABOUT IT
Suppose Star A is four times as luminous as Star B. How will
their apparent brightnesses compare if they are both the same
distance from Earth? How will their apparent brightnesses
compare if Star A is twice as far from Earth as Star B? Explain.
Document Summary
4p * distance2 use the standard units of luminosity are watts, the: assume that luminosity is known (standard candle), you can measure apparent brightness (b) and find the distance, b1/b2 = (d1/d2)2, a) cepheid stars: very luminous, pulsating. There is a relationship between period and luminosity. Fails at distances where you can"t make out individual stars: b) supernovae type ia: exploding white dwarves. You have mass falling into the white dwarf and if the mass of the white dwarf comes to exceed the critical limit, they explode. There is a relation between decay time and absolute luminosity of the supernova: low luminosity supernova fade away faster than high luminosity supernova. So, from the time it takes to fade away, you can measure the absolute luminosity: determine the distance by measuring the apparent luminosity & using formula above. Use this method to find distances of faraway galaxies (cid:24)(cid:20)(cid:28: used frequently because it is independent of other assumptions.